Cytochrome-b Sequence Variation in Water Shrews
(Sorex palustris) from Eastern and Western North America
Erin E. Mycroft, Aaron B.A. Shafer, and Donald T. Stewart
Northeastern Naturalist, Volume 18, Issue 4 (2011): 497–508
Full-text pdf (Accessible only to subscribers.To subscribe click here.)
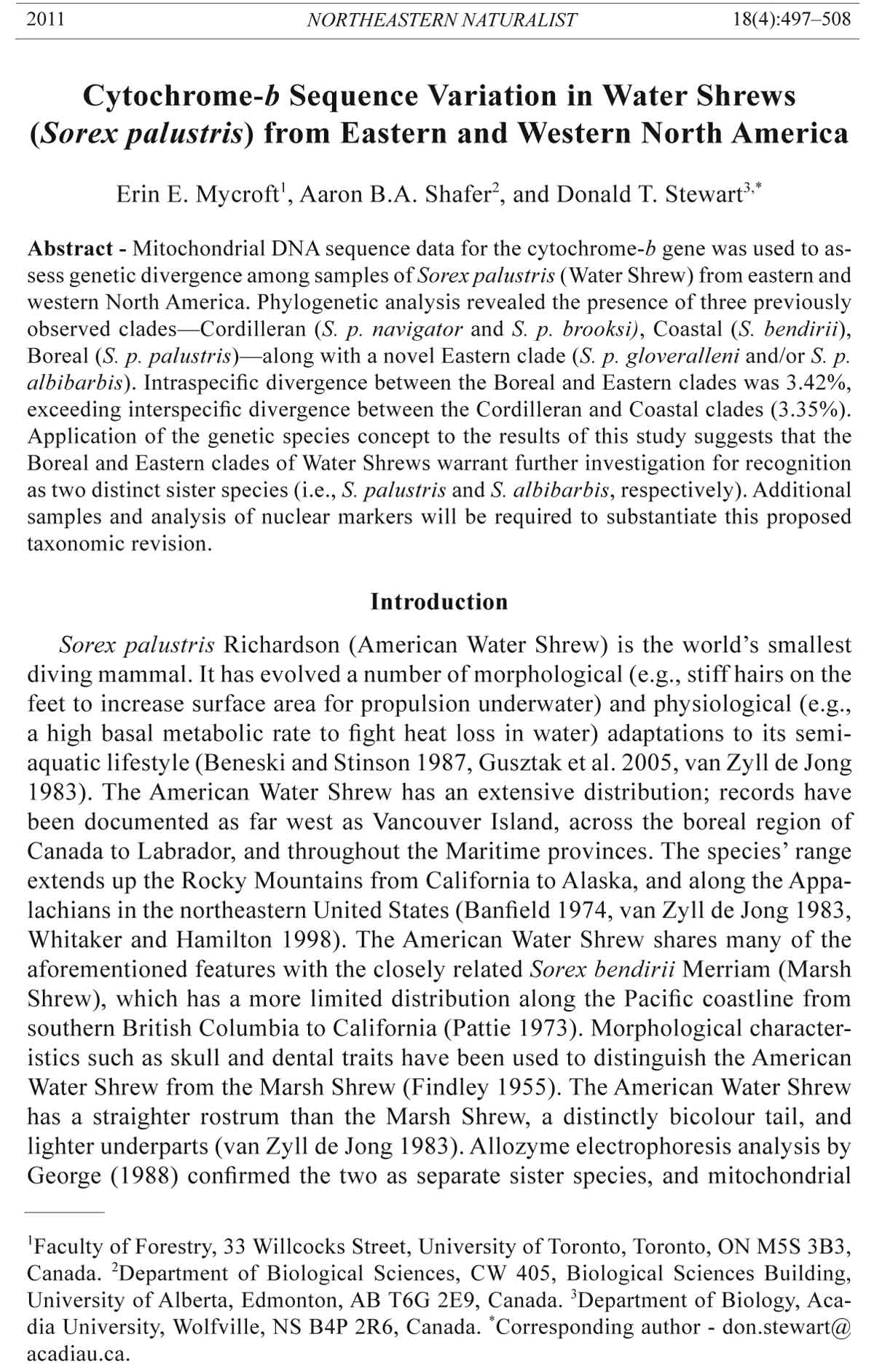
Access Journal Content
Open access browsing of table of contents and abstract pages. Full text pdfs available for download for subscribers.
Current Issue: Vol. 30 (3)
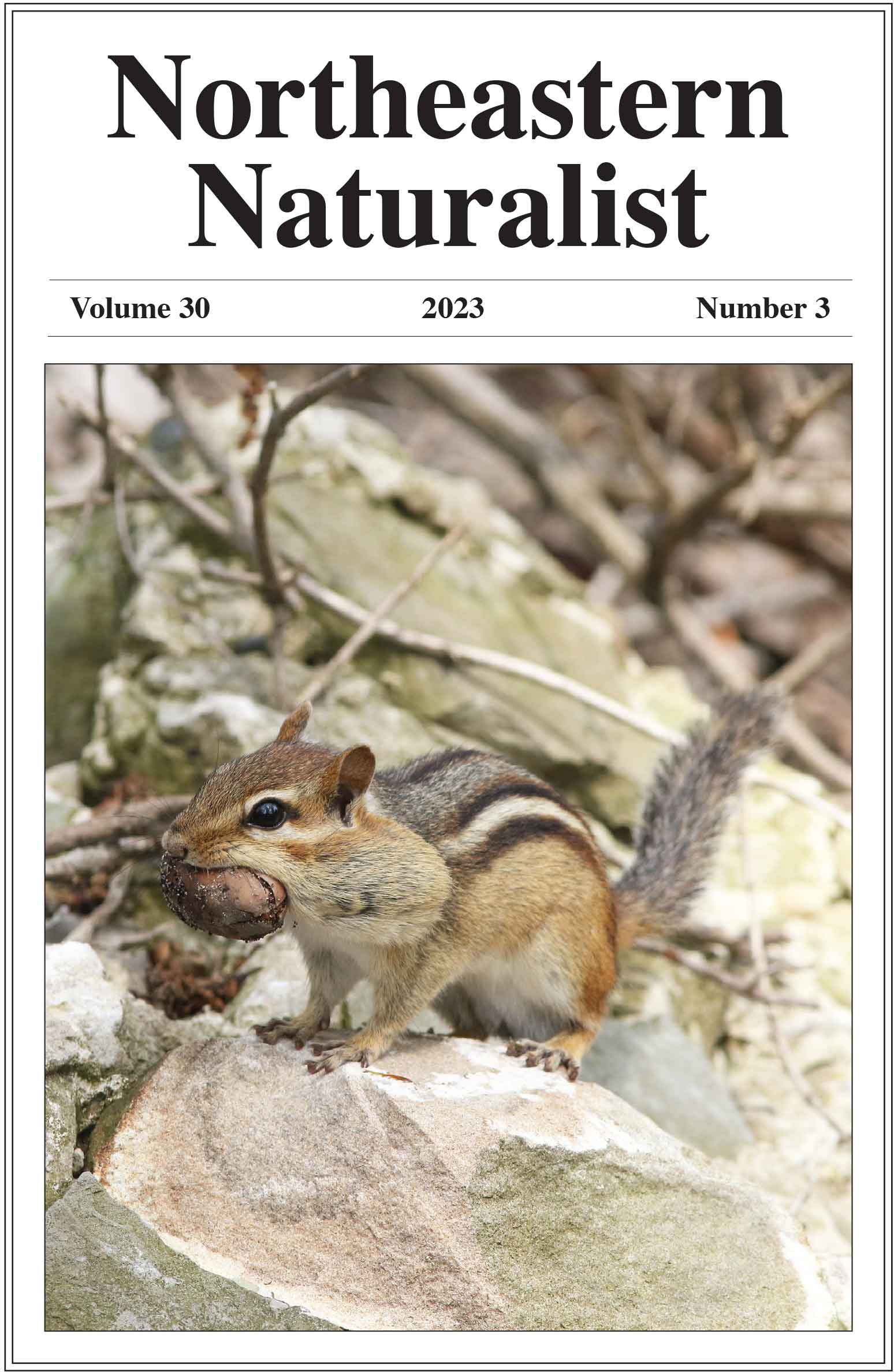
Check out NENA's latest Monograph:
Monograph 22
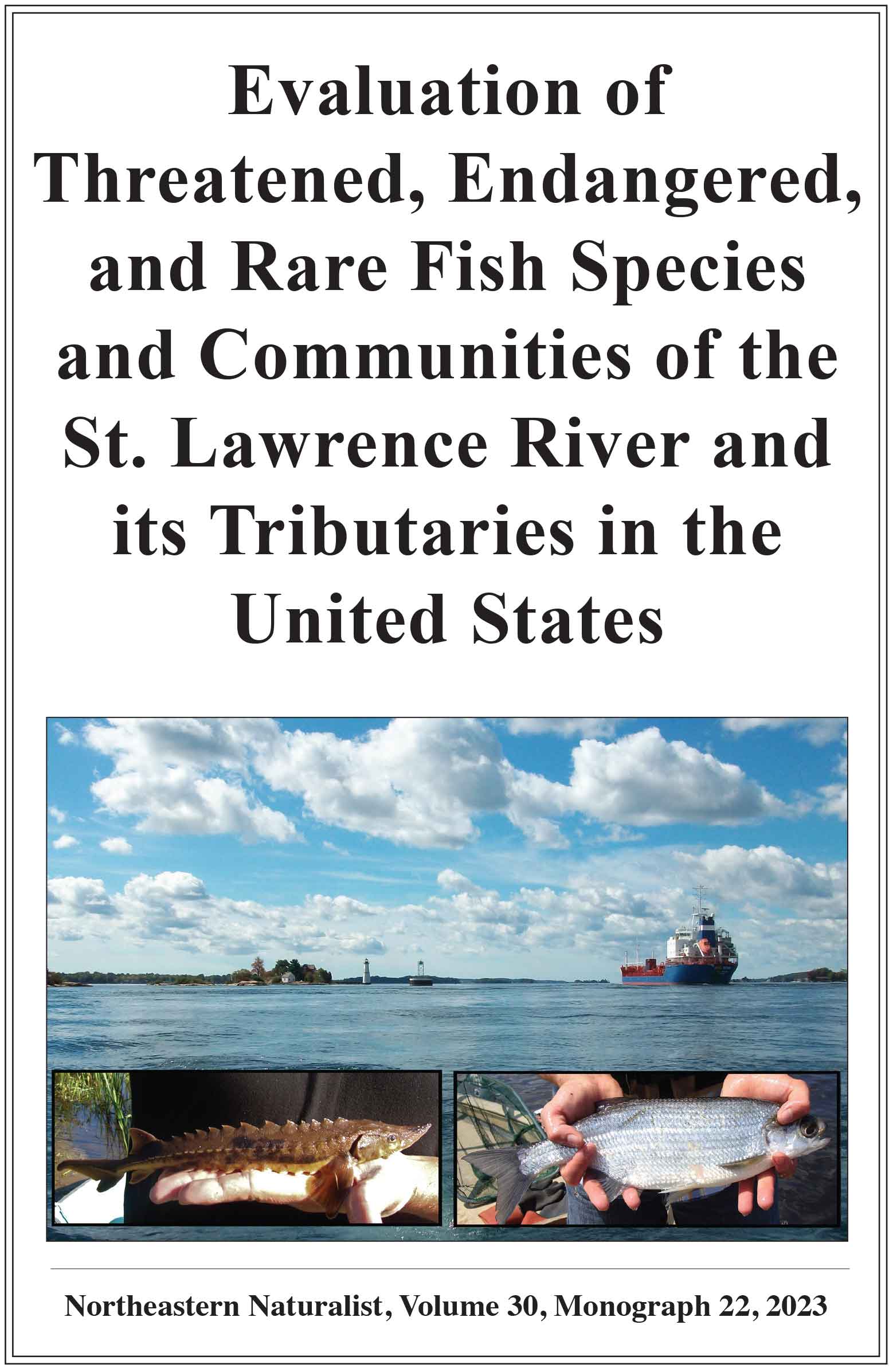
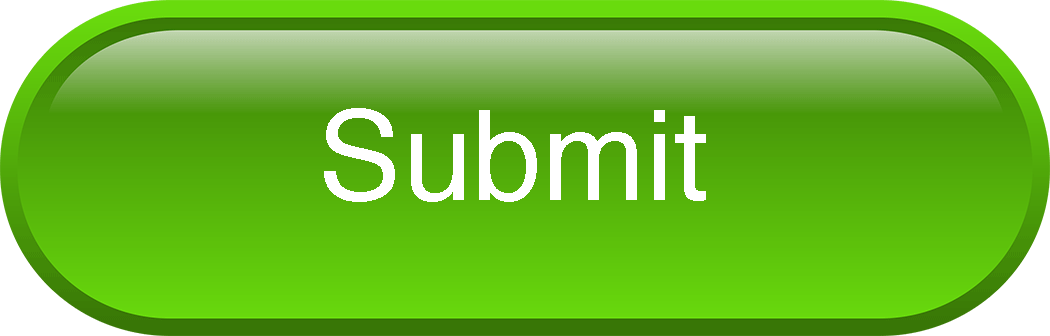
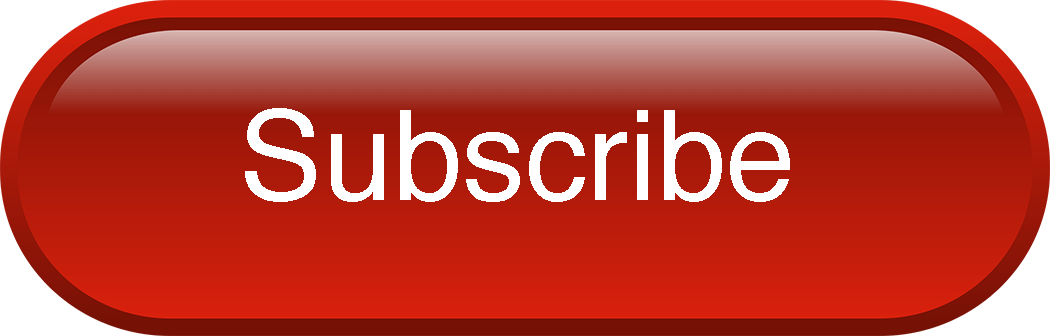
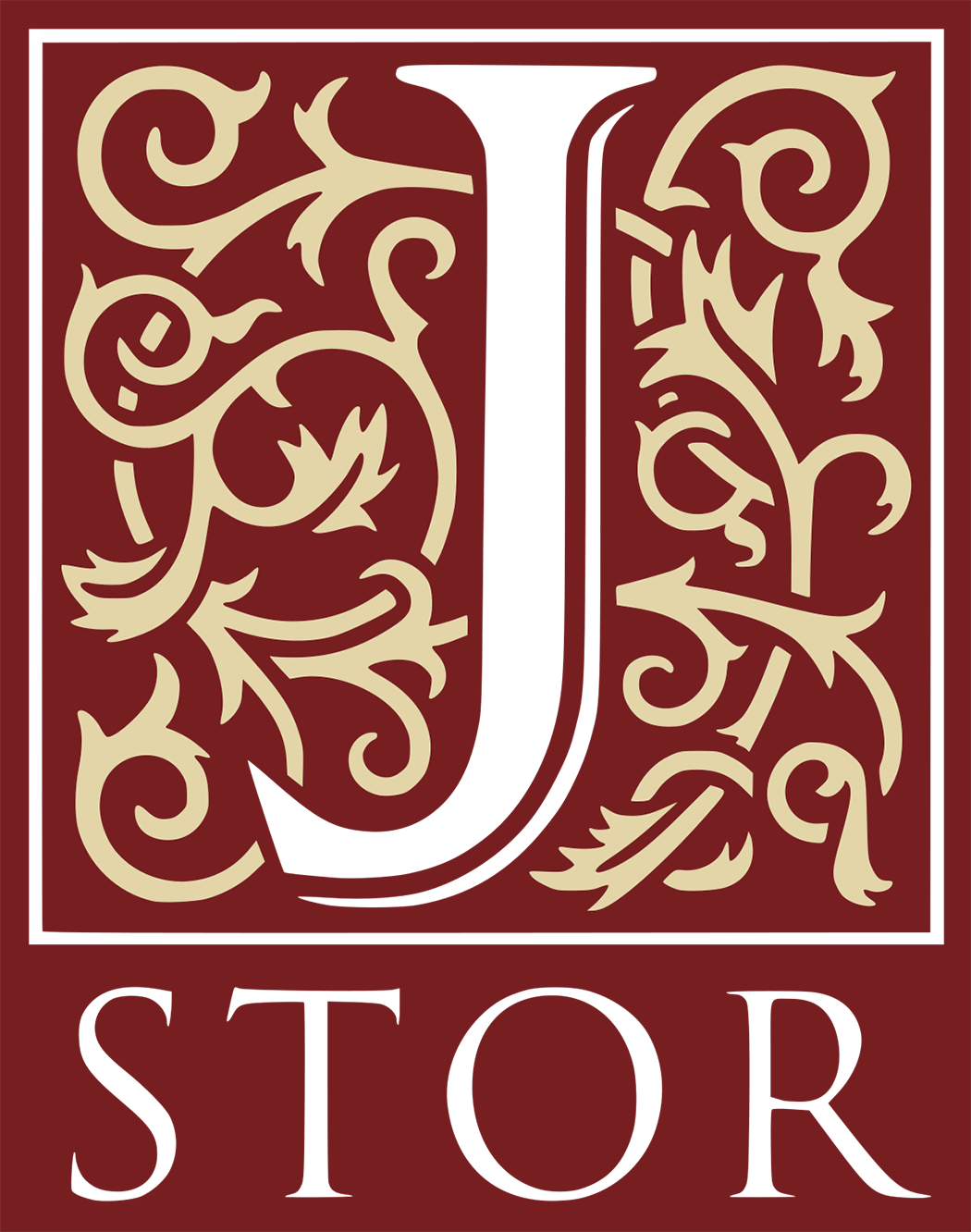
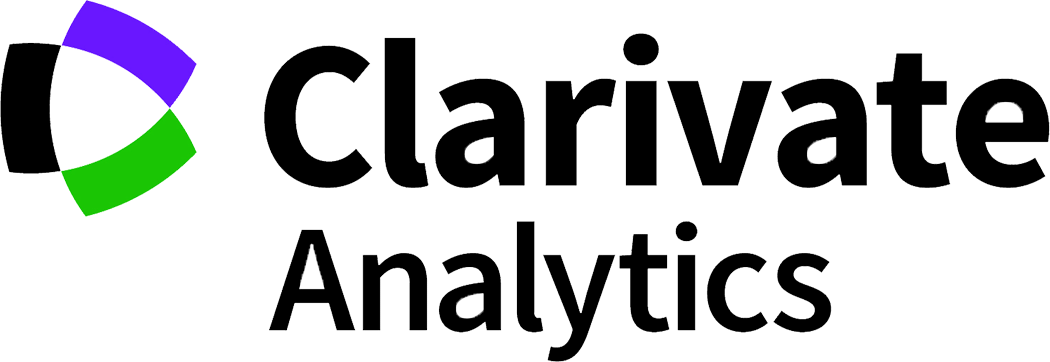
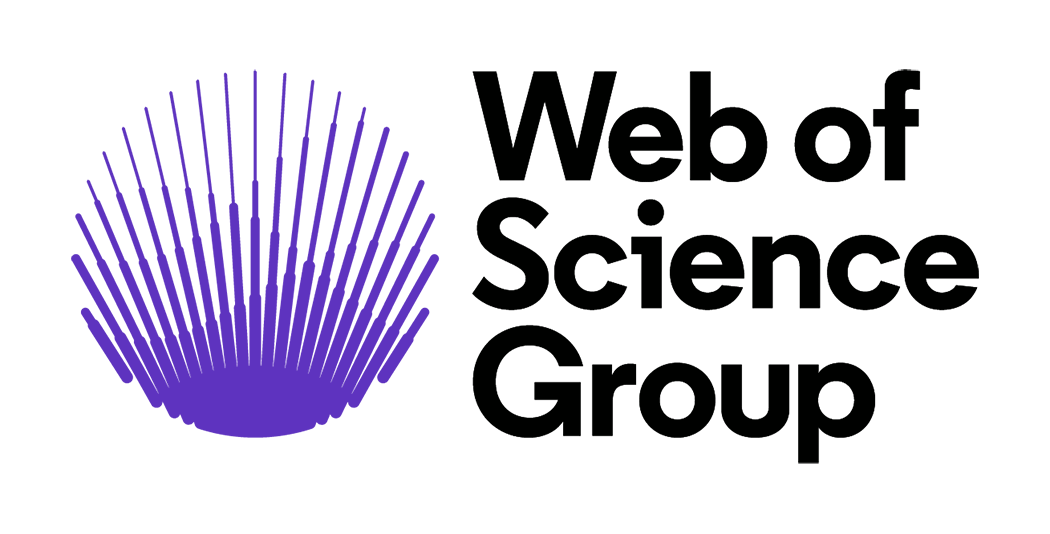
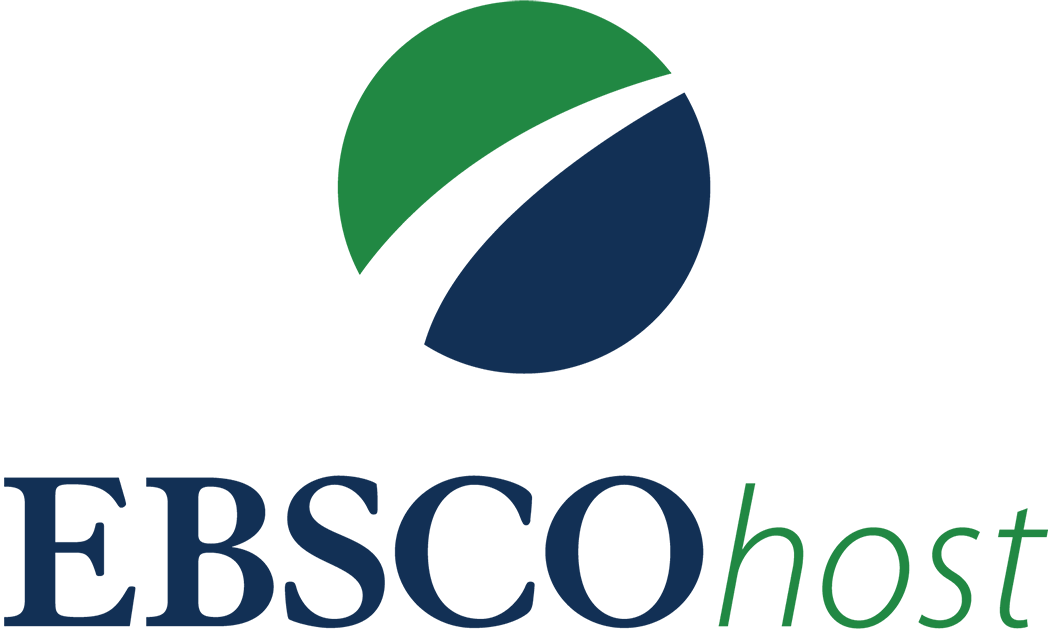
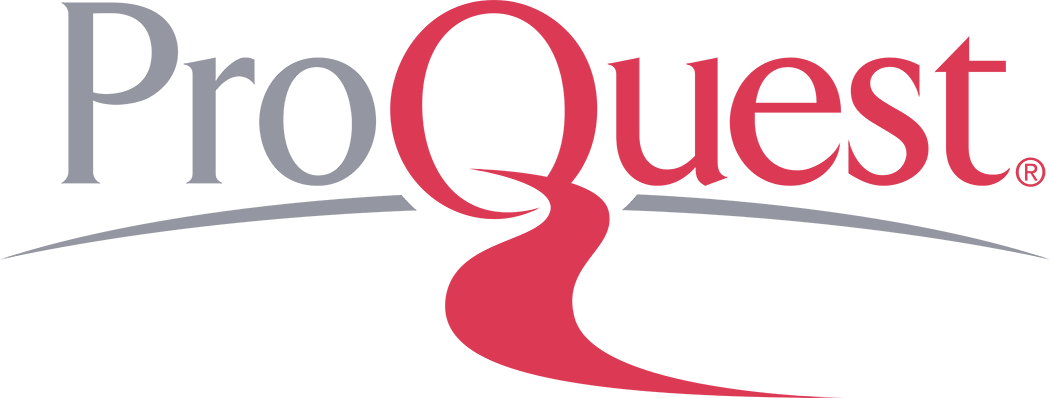
2011 NORTHEASTERN NATURALIST 18(4):497–508
Cytochrome-b Sequence Variation in Water Shrews
(Sorex palustris) from Eastern and Western North America
Erin E. Mycroft1, Aaron B.A. Shafer2, and Donald T. Stewart3,*
Abstract - Mitochondrial DNA sequence data for the cytochrome-b gene was used to assess
genetic divergence among samples of Sorex palustris (Water Shrew) from eastern and
western North America. Phylogenetic analysis revealed the presence of three previously
observed clades—Cordilleran (S. p. navigator and S. p. brooksi), Coastal (S. bendirii),
Boreal (S. p. palustris)—along with a novel Eastern clade (S. p. gloveralleni and/or S. p.
albibarbis). Intraspecific divergence between the Boreal and Eastern clades was 3.42%,
exceeding interspecific divergence between the Cordilleran and Coastal clades (3.35%).
Application of the genetic species concept to the results of this study suggests that the
Boreal and Eastern clades of Water Shrews warrant further investigation for recognition
as two distinct sister species (i.e., S. palustris and S. albibarbis, respectively). Additional
samples and analysis of nuclear markers will be required to substantiate this proposed
taxonomic revision.
Introduction
Sorex palustris Richardson (American Water Shrew) is the world’s smallest
diving mammal. It has evolved a number of morphological (e.g., stiff hairs on the
feet to increase surface area for propulsion underwater) and physiological (e.g.,
a high basal metabolic rate to fight heat loss in water) adaptations to its semiaquatic
lifestyle (Beneski and Stinson 1987, Gusztak et al. 2005, van Zyll de Jong
1983). The American Water Shrew has an extensive distribution; records have
been documented as far west as Vancouver Island, across the boreal region of
Canada to Labrador, and throughout the Maritime provinces. The species’ range
extends up the Rocky Mountains from California to Alaska, and along the Appalachians
in the northeastern United States (Banfield 1974, van Zyll de Jong 1983,
Whitaker and Hamilton 1998). The American Water Shrew shares many of the
aforementioned features with the closely related Sorex bendirii Merriam (Marsh
Shrew), which has a more limited distribution along the Pacific coastline from
southern British Columbia to California (Pattie 1973). Morphological characteristics
such as skull and dental traits have been used to distinguish the American
Water Shrew from the Marsh Shrew (Findley 1955). The American Water Shrew
has a straighter rostrum than the Marsh Shrew, a distinctly bicolour tail, and
lighter underparts (van Zyll de Jong 1983). Allozyme electrophoresis analysis by
George (1988) confirmed the two as separate sister species, and mitochondrial
1Faculty of Forestry, 33 Willcocks Street, University of Toronto, Toronto, ON M5S 3B3,
Canada. 2Department of Biological Sciences, CW 405, Biological Sciences Building,
University of Alberta, Edmonton, AB T6G 2E9, Canada. 3Department of Biology, Acadia
University, Wolfville, NS B4P 2R6, Canada. *Corresponding author - don.stewart@
acadiau.ca.
498 Northeastern Naturalist Vol. 18, No. 4
DNA cytochrome-b (mtDNA Cytb) sequence data also supported this relationship
(Demboski and Cook 2001, O’Neill et al. 2005).
At least nine subspecies of the American Water Shrew have been described
(van Zyll de Jong 1983). These subspecies do not differ significantly in size or
morphology, and were described mostly on the basis of color and tail characteristics.
Sorex palustris navigator is found in the southern Yukon Territory, British
Columbia, and in western Alberta. It has a distinct change in color between the
dorsal and ventral pelage and a bicolor tail. Sorex palustris brooksi is restricted
to Vancouver Island, and is also characterized by a black back and white hair on
the underside of the tail. These western subspecies appear to be derived from
a single refugium in the Pacific Northwest (Himes and Kenagy 2010), where
genetic clades do not appear to correspond to subspecies. Sorex palustris palustris
occurs in the boreal region of Canada from northeastern British Columbia
through to northern Ontario and Québec; it is characterized by a distinct change
in color between the dorsal and ventral area, and a bicolor tail. Sorex palustris
albibarbis occupies a range from southern Ontario and southern Québec through
to western New Brunswick; it does not possess as distinct a colour demarcation
or a bicolour tail. Sorex palustris gloveralleni is found in Prince Edward Island,
central and eastern New Brunswick, and Nova Scotia including Cape Breton. It
is described as having a pale grey pelage with a bicolor tail (van Zyll de Jong
1983). Subspecies S. p. hydrobadistes, S. p. turneri, S. p. labradorensis, and S. p.
punctulatus have been proposed to occur in Michigan and Wisconsin, Northern
Québec, Labrador, and the southern Appalachians, respectively (for detailed accounts
of these ranges see Banfield [1974] and Hall [1981]).
Variation in Cytb gene sequences of the American Water Shrew and the
Marsh Shrew from western North America was assessed by O’Neill et al.
(2005). Inter- and intra-specific divergence values and phylogenetic analyses
showed S. p. brooksi and S. p. navigator to be more closely related to S. b.
palmeri and S. b. bendirii than to another putative member of their own species,
S. p. palustris. The authors suggested that Water Shrew taxonomy be revised to
recognize the western Cordilleran subspecies as a distinct species, Sorex navigator
(Navigator Shrew; O’Neill et al. 2005). In the present study, geographic
variation of additional Water Shrew specimens from across North America was
investigated by examining genetic variation in the mtDNA Cytb gene of samples
obtained from both eastern and western regions. Based on our phylogenetic
and genetic distance analyses, we discuss possible taxonomic revisions to the
Water Shrew species complex.
Methods
Samples
Partial mitochondrial Cytb gene sequences (994 base pairs) were generated
from 10 specimens of Water Shrews from Alberta, Manitoba, Nova Scotia, and
New Brunswick. These samples correspond to the putative subspecies S. p.
palustris, S. p. albibarbis, and S. p. gloveralleni. Thirty-four additional Cytb
sequences, twenty-five of which are from Water or Marsh Shrews, were obtained
2011 E.E. Mycroft, A.B.A. Shafer, and D.T. Stewart 499
from Fumagalli et al. (1999), Demboski and Cook (2001), and O’Neill et al.
(2005) through the GenBank database (Table 1).
Mitochondrial DNA sequencing
Total DNA was extracted from frozen or ethanol-preserved tissue. Approximately
0.2 g of tissue per sample was used for DNA extraction (DNeasy
Table 1. GenBank accession numbers, location data, specimen/tissue numbers, articles referenced
from, and length of sequences for samples used in the analysis. Additional names not defined in the
text include Sorex vagrans Baird (Vagrant Shrew) and S. fumeus Miller (Smoky Shrew).
Specimen/ GenBank Phylogenetic
accession No. Location Article reference reference
S. p. navigator
AY954934 Yoho National Park, BC O’Neill et al. 2005 (BC1)
AY954936 Yoho National Park, BC O’Neill et al. 2005 (BC2)
AY954937 Cayoosh Creek, BC O’Neill et al. 2005 (BC3)
AY954933 Yoho National Park, BC O’Neill et al. 2005 (BC4)
AY954935 Yoho National Park, BC O’Neill et al. 2005 (BC5)
AY954932 Yoho National Park, BC O’Neill et al. 2005 (BC6)
AJ000448 Salt Lake County, UT Fumagalli et al.1999 (UT1)
AJ000449 Watson Lake, YT Fumagalli et al.1999 (YK1)
AF238033 Petersburg Quad, AK Demboski and Cook 2001 (AK1)
S. p. brooksii
AY954926 Hamilton Creek, Vancouver O’Neill et al. 2005 (BC1)
Island, BC
AY954927 Hamilton Creek, Vancouver O’Neill et al. 2005 (BC2)
Island, BC
AY954931 Lower Lost Shoe Creek, O’Neill et al. 2005 (BC3)
Vancouver Island, BC
AY954928 Lowry Lake, Vancouver Island, BC O’Neill et al. 2005 (BC4)
AY954929 Lowry Lake, Vancouver Island, BC O’Neill et al. 2005 (BC5)
AY954930 Lowry Lake, Vancouver Island, BC O’Neill et al. 2005 (BC6)
S. b. bendirii
AY954944 Sumas Mountain, BC O’Neill et al. 2005 (BC1)
AY954945 Sumas Mountain, BC O’Neill et al. 2005 (BC2)
AY954946 Seymour River, BC O’Neill et al. 2005 (BC3)
AY954947 Aldergrove, BC O’Neill et al. 2005 (BC4)
S. p. palustris
AY954938 Calling Lake, AB O’Neill et al. 2005 (AB1)
AY954940 Calling Lake, AB O’Neill et al. 2005 (AB2)
AY954941 Calling Lake, AB O’Neill et al. 2005 (AB3)
AY954942 Calling Lake, AB O’Neill et al. 2005 (AB4)
AY954939 Calling Lake, AB O’Neill et al. 2005 (AB5)
AY954943 Lac La Biche, AB O’Neill et al. 2005 (AB6)
DQ788800 Ototoks, AB This study (AB7)
DQ995274 Caddy Lake, MB This study (MB1)
DQ995275 Piney, MB This study (MB2)
S. p. albibarbis
DQ995279 Edmundston, NB This study (NB1)
DQ995280 Edmundston, NB This study (NB2)
500 Northeastern Naturalist Vol. 18, No. 4
Tissue Kit, QIAGEN). Samples were diluted to 25 ug DNA/ml in preparation
for polymerase chain reaction (PCR). Amplification of the Cytb gene was accomplished
with a PCR reaction using L14841 (Kocher et al. 1989) or L14724,
and H15915 primers (Irwin et al. 1991). PCR reactions were 25 ul each, consisting
of 12.45 ul ultra-pure water, 2.5 ul 10x Buffer, 2.5 ul L14724 primer
[10 pmol], 2.5 ul H15915 primer [10 pmol], 2.5 ul dNTPs [8mM], 0.3 ul Taq
polymerase [5 U/ul] (Promega), 1.25 ul MgCl2 [25 mM], and 1 ul of [25 ug/
ml] DNA template. The PCR program was an initial 94 °C for 3 min, followed
by 35 cycles of 94 °C for 45 s, 50 °C for 45 s, and extension at 72 °C for 1
min, concluding with 72 °C for 5 min. PCR products were purified using the
QIAquick Gel Purification Kit (QIAGEN). Double-stranded sequencing was
performed at the Florida State University DNA Sequencing Facility (Tallahassee,
FL) using an Applied Biosystems 3100 Genetic Analyzer. Mitochondrial
Cytb sequences were aligned with the ClustalW algorithm (Thompson et al.
1994) and edited using BioEdit v.7.0.5.3 (Hall 1999).
Data analysis
For comparison with previous studies, the traditional neighbor-joining (NJ)
method of analysis was performed using the Kimura 2-parameter model of substitution
in MEGA (Kumar et al. 2004). Maximum parsimony (MP) analysis
was conducted using PAUP v.4.0b10 (Swofford 2002). A heuristic search was
conducted on all data sets, with 10 replicates of the addition sequence, and equal
character weighting. A tree bisection-reconnection (TBR) branch-swapping
Table 1, continued.
Specimen/ GenBank Phylogenetic
accession No. Location Article reference reference
S. p. gloveralleni
DQ995276 Mount Martock, NS This study (NS1)
DQ995277 Waverley, NS This study (NS2)
DQ788802 Cole Harbour, NS This study (NS3)
DQ788801 Cape Breton, NS This study (NS4)
DQ995278 Cape Breton, NS This study (NS5)
S. monticolus
AJ000451 Tillamook County, OR Fumagalli et al.1999 (OR1)
AJ000450 Tillamook County, OR Fumagalli et al.1999 (OR2)
S. fumeus
AJ000462 Edmundston, NB Fumagalli et al.1999 (NB1)
AJ000461 Edmundston, NB Fumagalli et al.1999 (NB2)
S. hoyi
AJ000460 Ogilvie Mountains, YT Fumagalli et al.1999 (YK1)
AF238040 Hughes Quad, AK Demboski and Cook 2001 (AK1)
S. vagrans
AJ000455 Sagehen Creek, CA Fumagalli et al.1999 (CA1)
AJ000454 Sagehen Creek, CA Fumagalli et al. 1999 (CA2)
S. araneus
AY936837 Sweden Andersson et al. 2005
2011 E.E. Mycroft, A.B.A. Shafer, and D.T. Stewart 501
algorithm was also implemented. For both NJ and MP analyses, the robustness
of the resulting tree was evaluated by performing 1000 bootstrap replicates.
The appropriate model for the maximum likelihood (ML) analysis was
determined with MODELTEST (Posada and Crandall 1998), with the model
TrN+G chosen as the best-fit model of nucleotide substitution. Taxa were
added to the tree using a random addition sequence (x10), and the TBR branchswapping
algorithm was employed. Due to the computational time required,
the robustness of the ML tree topology was tested in PAUP v.4.0b10 with 100
bootstrap replicates, and the number of trees saved per replicate was reduced
to one (MAXTREE = 1). The Bayesian analysis was conducted using MrBayes
v.3.1 (Huelsenbeck and Ronquist 2001). Codon positions were treated separately
with models determined from MRMODELTEST (Nylander 2004), as the
frequency of substitution varies greatly among codon positions in Cytb (Irwin
et al. 1991). Each position (1st, 2nd, and 3rd, which had the models HKY+G,
HKY+I, and HKY+I+G, respectively) were unlinked, and were allowed to
evolve separately. The Markov chain Monte Carlo algorithm was run with four
chains and sampled every 1000 generations until the average standard deviation
of split frequencies stabilized at less than 0.01. All chains prior to the stabilization
of likelihood values were removed as a burn-in, and the remainder were used
to construct a 50% consensus tree.
Pairwise genetic distances were determined with MEGA using Kimura’s
two-parameter model with complete deletion (Table 2). This model was chosen
to enable comparison of results with those of other studies (e.g., Demboski and
Cook 2001, 2003; Fumagalli et al. 1999; O’Neill et al. 2005).
Table 2. Percent divergence in Cytochrome-b sequences between the four major clades (consisting
of S. palustris and S. bendirii) and outgroups using the Kimura 2-parameter model and complete
deletion. S. h. = Sorex hoyi, S. f. = S. fumeus, S. m. = S. monticolus, and S. v. = S. vagrans.
Cordilleran Coastal Boreal Eastern S. h. S. f. S. m. S. v.
Group 1 “Cordilleran”
(S. p. navigator/ - - - - - - - -
S. p. brooksi)
Group 2 “Coastal”
(S. bendirii) 3.35 - - - - - - -
Group 3 “Boreal”
(S. p. palustris) 7.21 6.61 - - - - - -
Group 4 “Eastern”
(S. p. albibarbis/ 6.12 6.48 3.42 - - - - -
S. p. gloveralleni)
S. hoyi 13.35 11.96 12.91 12.18 - - - -
S. fumeus 13.36 12.84 13.09 13.16 14.15 - - -
S. monticolus 5.09 4.41 7.01 5.82 12.47 13.16 - -
S. vagrans 8.25 7.13 8.61 7.90 12.18 13.24 7.90 -
S. araneus 15.96 16.33 17.00 16.41 17.39 18.59 15.52 17.50
502 Northeastern Naturalist Vol. 18, No. 4
Figure 1 (opposite page). Phylogenetic relationships for members of the genus Sorex, inferred
from 994bp of mtDNA Cytb sequence data, rooted with Sorex araneus L. (European
Common Shrews) as an outgroup. Bootstrap support values are for the neighbor-joining
maximum-parsimony (above the branch) and maximum-likelihood (below the branch),
respectively. For the first two methods, 1000 bootstrap replicates were performed; for
maximum-likelihood analysis, 100 bootstrap replicates were used. For clarity, only bootstrap
values of major clades are displayed on branches. Identifiers in brackets correspond
to Genbank accession numbers and can be found in Table 1.
Results
Phylogenetic analysis
Neighbor-joining, maximum parsimony, maximum likelihood, and Bayesian
methods of analysis produced near-identical trees with similar support values
for most clades (see Figs. 1 and 2). Four distinct clades were evident: a “Cordilleran”
clade (S. p. navigator and S. p. brooksi), a “Coastal” clade (S. b. bendirii),
a “Boreal” clade (S. p. palustris), and an “Eastern” clade (S. p. gloveralleni,
S. p. albibarbis). The subspecies contained within the “Eastern” clade have been
inferred using putative geographical ranges (Banfield 1974) and are not definitive.
The “Cordilleran” and “Coastal” clades form one group, and the “Boreal”
and “Eastern” clades form another. Sorex monticolus Merriam (Montane Shrew)
Cytb sequences formed a sister-group to the combined “Cordilleran” and “Coastal”
clades of Water Shrews. The neighbor-joining tree had high bootstrap support
values, ranging from 99–100% for each major clade, as did the maximumparsimony
tree (95–100%; Fig. 1). For the Bayesian analysis, we ran 500,000
generations with the first 10% removed as a burn-in (based on plot of likelihood
values). The average standard deviation of split frequencies was below 0.01, and
the potential scale reduction factors were ≈1, indicative of convergence. Every
major clade was highly supported, with posterior probabilities ranging from
0.97–1.00 (Fig. 2).
Sequence divergence values
Sequence divergence values between the four major Water Shrew clades (i.e.,
“Cordilleran”, “Coastal”, “Boreal”, and “Eastern”) and the outgroups are presented
in Table 2. The level of divergence between the “Boreal” and “Eastern” clades
consisting of S. palustris (3.42%) was greater than that between the “Cordilleran”
and “Coastal” clades (3.35%). Distance calculations grouping “Cordilleran” and
“Coastal” clades together as one group and “Boreal” and “Eastern” clades as another
revealed a divergence value of 6.70%.
Discussion
In the genus Sorex, interspecific distance estimates for the Cytb gene of
selected species range from 0.7% to 9.3% (Stewart et al. 2002, 2003). In this
study, intraspecific distance between the “Boreal” and “Eastern” clades of the
American Water Shrew was found to be 3.42%. This value falls within the range
2011 E.E. Mycroft, A.B.A. Shafer, and D.T. Stewart 503
504 Northeastern Naturalist Vol. 18, No. 4
Figure 2. Bayesian tree for members of the genus Sorex, inferred from 994bp of mtDNA
Cytb sequence data, rooted with European Common as an outgroup. Numbers correspond
to posterior probabilities, using the model TrN+G. Identifiers in brackets correspond to
Genbank accession numbers and can be found in Table 1.
of interspecific divergence in the genus Sorex, and in accordance with the genetic
species concept (sensu Baker and Bradley 2006), suggests these two clades may
be distinct species. For example, divergence between two putative species of
S. hoyi Baird (American Pygmy Shrew) and S. thompsoni Baird (Thompson’s
Pygmy Shrew) is 3.3% (Stewart et al. 2003), while the Marsh Shrew and the
Navigator Shrew Cytb sequences differ by approximately 3.1% (O’Neill et al.
2005) to 3.35% (this study). Furthermore, Shafer and Stewart (2007) found evidence
for a distinct eastern group of Water Shrews. While our data suggest that
the “Boreal” and “Eastern” clades of Water shrews may indeed warrant separate
2011 E.E. Mycroft, A.B.A. Shafer, and D.T. Stewart 505
species status, further evidence from a nuclear locus would be a valuable addition
to accurately delineate species.
A “genetic” species has been defined as “a group of genetically compatible
interbreeding natural populations that is genetically isolated from other such
groups” (Baker and Bradley 2006). Some mammalian species, such as those belonging
to the genus Sorex, are difficult to distinguish based on morphology alone
(Baker and Bradley 2006). To develop a genetic distance metric that could be
used to identify potentially cryptic species, Bradley and Baker (2001) analyzed
levels of Cytb sequence variation required for speciation in 4 genera of rodents
and 7 genera of bats; the authors concluded that a genetic distance of >11% indicates
definite interspecific variation; values of 2% to 11% warrant additional
investigation, and values of <2% likely indicate intraspecific or population-level
variation. The precise “threshold” divergence required for speciation may vary
depending on the group being studied (Baker and Bradley 2006, Bradley and
Baker 2001). Nonetheless, there is increasing evidence that the general utility of
mitochondrial “DNA Barcoding” for identifying species divergence thresholds
reflects the intense co-evolutionary selective pressures on mitochondrial genes
and nuclear genes to produce the functional components for cellular respiration
(Lane 2009). If the mitochondrial and nuclear encoded subunits do not work well
together (as is increasingly likely with increasing molecular divergence), then
respiration will be less efficient or fail altogether.
The major inference to be drawn from the phylogenetic and distance analyses
presented here is that the “Eastern” clade of Water Shrews may be a distinct species
from the “Boreal” clade. Under this division, the “Boreal” clade would represent
S. palustris, while the “Eastern” clade, which contains representative samples from
two putative subspecies, S. p. albibarbis and S. p. gloveralleni, would be renamed
S. albibarbis Cope, which has historical priority (Cope 1862). A tentative common
name for this taxon could be the White-bearded Water Shrew. Additional nuclear
data and sampling are required prior to a full taxonomic revision.
The phylogenetic affinities of the remaining subspecies in eastern North
America (namely S. p. hydrobadistes, S. p. labradorensis, S. p. turneri, and
S. p. punctulatus) will also need to be directly assessed before they are assigned
to either S. palustris or S. albibarbis. Two scenarios are most probable. First,
subspecies distributed throughout the boreal forest (i.e., S. p. palustris, S. p.
turneri, and S. p. labradorensis), and those present in the Acadian and Great
Lakes-St. Lawrence forest zones as well as those in the Appalachian Mountains
(i.e., S. p. gloveralleni, S. p. albibarbis, S. p. hydrobadistes, and S. p. punctulatus)
will cluster together with either S. palustris or S. albibarbis, respectively.
Alternatively, the St. Lawrence River and Great Lakes waterway may
constitute a biogeographic barrier that separates two distinctive mitochondrial
clades (e.g., S. palustris to the north and S. albibarbis to the south and east), in
which case any putative subspecific designations may be need to be revisited.
The phylogenetic position of the two Edmundston, NB, samples (see Fig. 1) is
of particular interest in this context. One of these samples (NB2) is embedded
506 Northeastern Naturalist Vol. 18, No. 4
within a clade of Nova Scotia sequences and the other (NB1) forms a sister sequence
to this clade. This pattern suggests that the area around Edmundston in
northwestern New Brunswick is home to Water Shrews with two distinct, albeit
very closely related, haplotypes (0.7% sequence divergence). This is the same
pattern that is observed within S. cinereus Kerr (Masked Shrews) in eastern
Canada. Specifically, Stewart and Baker (1997) found that northwestern New
Brunswick was a zone of secondary contact between two mitochondrial clades
within Masked Shrews: one primarily a maritime clade (corresponding to the
Acadian forest region), and one primarily a Québec and Ontario clade (corresponding
more closely to the Great Lakes-St. Lawrence forest region). Given
that Water Shrews and Masked Shrews have similar distributions, it would not
be surprising that they share similar biogeographic histories. The Wisconsin
glacial events that likely lead to the divergence of the distinct mitochondrial
clades within Masked Shrews also likely shaped the pattern of molecular divergence
within Water Shrews.
In summary, further work is needed to clarify the phylogenetic relationships,
taxonomic status, historical biogeography, differences in natural history, and
conservation status of all American Water and Marsh shrews. The application
of novel species concepts, (e.g., the genetic species concept) can be of enormous
practical benefit to the study of shrews or other small mammals that are
either morphologically non-descript or challenging to maintain in captivity (see
Gusztak and Campbell 2004). More voucher specimens of Water Shrews need to
be examined both morphologically and genetically from across the full species
range. Efforts should also be focused on geographically intermediate areas of
genetically distinct clades to identify potential zones of introgression.
Acknowledgments
Samples from Manitoba were kindly supplied by K.L. Campbell at the University of
Manitoba. Funding for this research was provided by a Natural Sciences and Engineering
Council of Canada Discovery Grant to D.T. Stewart (217175). Thanks also to the Biology
“Block program” at Acadia University, funded in part by the Office of the Dean, Faculty
of Pure and Applied Science, for the unique opportunity for undergraduate students to
become engaged in an intensive learning and research environment.
Literature Cited
Andersson, A.C., C. Alström-Rapaport, and K. Fredga. 2005. Lack of mitochondrial
DNA divergence between chromosome races of the Common Shrew, Sorex araneus,
in Sweden. Implications for interpreting chromosomal evolution and colonization
history. Molecular Ecology 14:2703–2716.
Baker, R.J., and R.D. Bradley. 2006. Speciation in mammals and the genetic species
concept. Journal of Mammalogy 87:643–662.
Banfield, A.W.F. 1974. The Mammals of Canada. University of Toronto Press, Toronto,
ON, Canada.
Beneski, J.T., and D.W. Stinson. 1987. Sorex palustris. Mammalian Species 296:1–6.
Bradley, R.D., and R.J. Baker. 2001. A test of the genetic species concept: Cytochrome-b
sequences and mammals. Journal of Mammalogy 82:960–973.
2011 E.E. Mycroft, A.B.A. Shafer, and D.T. Stewart 507
Cope, E.D. 1862. On Neosorex albibarbis. Proceedings of the Academy of Natural Sciences,
Philadelphia. 14:188–189.
Demboski, J.R., and J.A. Cook. 2001. Phylogeography of the Dusky Shrew, Sorex monticolus
(Insectivora, Soricidae): Insight into deep and shallow history in northwestern
North America. Molecular Ecology 10:1227–1240.
Demboski, J.R., and J.A. Cook. 2003. Phylogenetic diversification within the Sorex cinereus
complex (Insectivora: Soricidae). Journal of Mammalogy 84:144–158.
Findley, J.S. 1955. Taxonomy and distribution of some American shrews. University of
Kansas Publications, Museum of Natural History 7:613–618.
Fumagalli, L., P. Taberlet, D.T. Stewart, L. Gielly, J. Hausser, and P. Vogel. 1999. Molecular
phylogeny and evolution of Sorex shrews (Soricidae: Insectivora) inferred
from mitochondrial DNA sequence data. Molecular Phylogenetics and Evolution
11:222–235.
George, S.B. 1988. Systematics, historical biogeography, and evolution of the genus
Sorex. Journal of Mammalogy 69:443–461.
Gusztak, R.W., and K.L. Campbell. 2004. Growth, development, and maintenance of
American Water Shrews (Sorex palustris) in captivity. Mammal Study 9:65–72.
Gusztak, R.W., R.A. MacArthur, and K.L. Campbell. 2005. Bioenergetics and thermal
physiology of American Water Shrews. Journal of Comparative Physiology B
175:87–95.
Hall, E.R. 1981. The Mammals of North America. Volume 1. John Wiley and Sons, New
York, NY.
Hall, T.A. 1999. BioEdit: A user-friendly biological sequence alignment editor and analysis
program for Windows 95/98/NT. Nucleic Acids Symposium Series 41:95–98.
Himes, C.M., and G. J. Kenagy. 2010. Influence of montane isolation and refugia on
population structure of Sorex palustris in western North America. Journal of Mammalogy
91:1000–1010.
Huelsenbeck J.P., and F. Ronquist. 2001. MRBAYES: Bayesian inference of phylogenetic
trees. Bioinformatics 17:754–755.
Irwin, D.M., T.D. Kocher, and A.C. Wilson. 1991. Evolution of the cytochrome-b gene
of mammals. Journal of Molecular Evolution 32:128–144.
Kocher, T.D., W.K. Thomas, A. Meyer, S.V. Edwards, S. Paabo, F.X. Villablanca, and
A.C. Wilson. 1989. Dynamics of mitochondrial DNA evolution in animals: Amplification
and sequencing with conserved primers. Proceedings of the National Academy of
Sciences of the United States of America. 86:6196–6200.
Kumar, S., K. Tamura, and M. Nei. 2004. MEGA3: Integrated software for Molecular
Evolutionary Genetics Analysis and sequence alignment. Briefings in Bioinformatics
5:150–163.
Lane, N. 2009. On the origin of barcodes. Nature 462:272–274.
Nylander, J.A.A. 2004. MrModeltest v2. Program distributed by the author. Evolutionary
Biology Centre, Uppsala University, Uppsala, Sweden.
O’Neill, M.B., D.W. Nagorsen, and R.J. Baker. 2005. Mitochondrial DNA variation in water
shrews (Sorex palustris, Sorex bendirii) from western North America: Implications
for taxonomy and phylogeography. Canadian Journal of Zoology 83:1469–1475.
Pattie, D. 1973. Sorex bendirii. Mammalian Species 27:1–2.
Posada, D., and K.A. Crandall. 1998. Modeltest: Testing the model of DNA substitution.
Bioinformatics 14:817–818.
508 Northeastern Naturalist Vol. 18, No. 4
Shafer, A.B.A., and D.T. Stewart. 2007. Phylogenetic relationships of Nearctic shrews
within the genus Sorex (Insectivora, Soricidae) inferred from combined cytochrome-b
and inter-SINE fingerprint data using Bayesian analysis. Molecular Phylogenetics and
Evolution 44:192–203.
Stewart, D.T., and A.J. Baker. 1997. A phylogeny of some taxa of Masked Shrews (Sorex
cinereus) based on mitochondrial DNA D-loop sequences. Journal of Mammalogy
78:361–376.
Stewart, D.T., N.D. Perry, and L. Fumagalli. 2002. The Maritime Shrew, Sorex maritimensis
(Insectivora: Soricidae): A newly recognized, Canadian endemic. Canadian
Journal of Zoology 80:94–99.
Stewart, D.T., M. McPherson, M.J.R. Robichaud, and L. Fumagalli. 2003. Are there two
species of pygmy shrews (Sorex)? Revisiting the question using DNA sequence data.
Canadian Field-Naturalist 117:82–88.
Swofford, D.L. 2002. PAUP* 4.0b10: Phylogenetic Analysis Using Parsimony (and other
methods). Sinauer Associates, Inc., Sunderland, MA.
Thompson, J.D., D.G. Higgins, and T.J. Gibson. 1994. CLUSTAL W: Improving the
sensitivity of progressive multiple sequence alignment through sequence weighting,
positions-specific gap penalties, and weight matrix choice. Nucleic Acids Research
22:4673–4680.
van Zyll de Jong, C.G. 1983. Handbook of Canadian Mammals. Volume 1. Marsupials
and Insectivores. National Museums of Canada, Ottawa, ON, Canada.
Whitaker, J.O., Jr., and W.J. Hamilton, Jr. 1998. Mammals of the Eastern United States.
Cornell University Press, Ithaca, NY.