All Taxa Biodiversity Inventory Survey of Select Soil and Plant Ecological Parameters Associated with Rhododendron
Decline in the Great Smoky Mountains and Surrounding
Areas
Richard Baird, Alicia Wood-Jones, Jac Varco, Clarence Watson, William Starrett, Glenn Taylor, and Kristine Johnson
Southeastern Naturalist, Volume 12, Issue 4 (2013): 703–722
Full-text pdf (Accessible only to subscribers.To subscribe click here.)
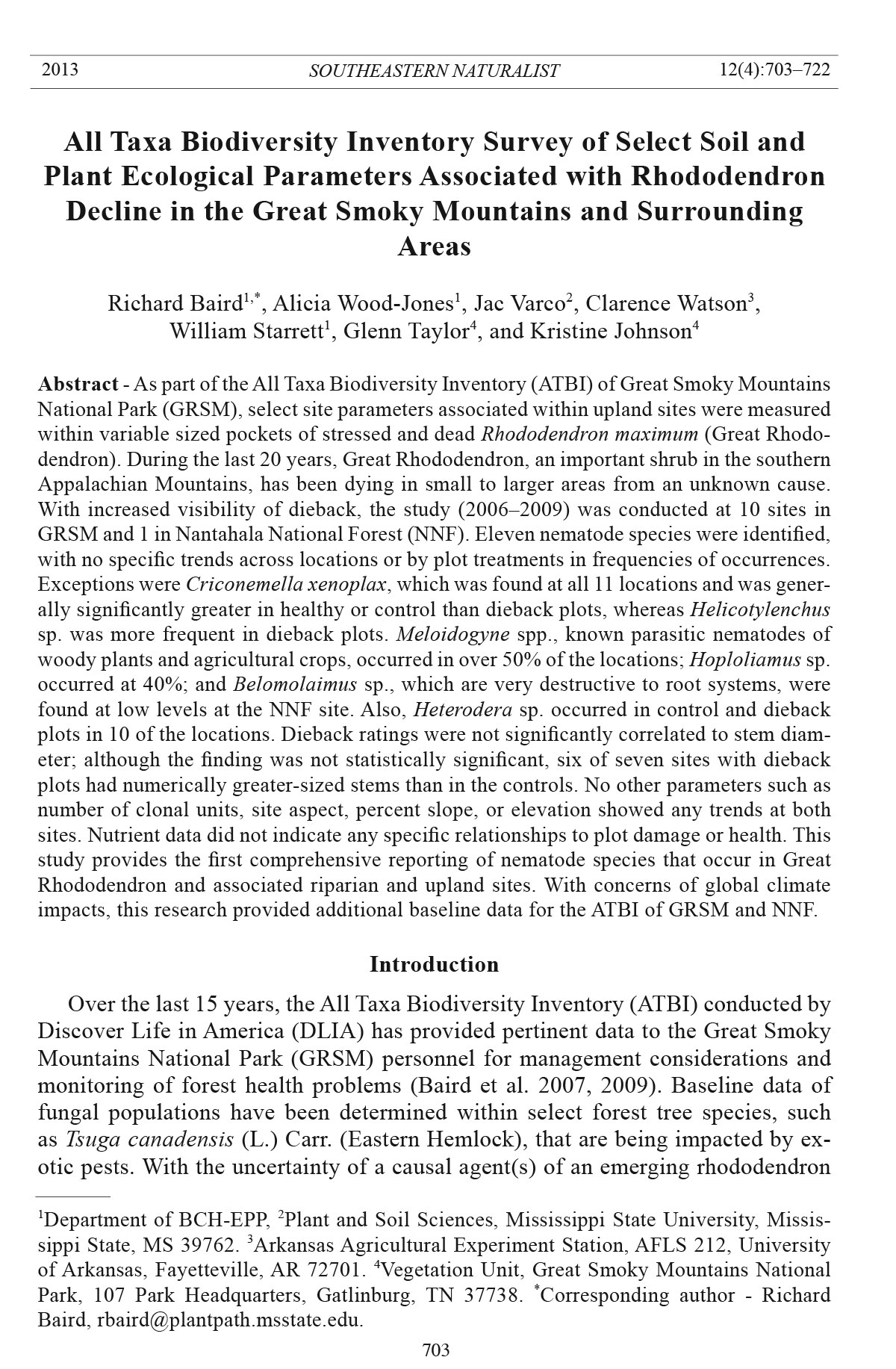
703
R. Baird, A. Wood-Jones, J. Varco, C. Watson, W. Starrett, G. Taylor, and K. Johnson
22001133 SOUTSoHuEthAeSaTsEteRrnN NNaAtTurUaRliAstLIST 1V2o(4l.) :1720,3 N–7o2. 24
All Taxa Biodiversity Inventory Survey of Select Soil and
Plant Ecological Parameters Associated with Rhododendron
Decline in the Great Smoky Mountains and Surrounding
Areas
Richard Baird1,*, Alicia Wood-Jones1, Jac Varco2, Clarence Watson3,
William Starrett1, Glenn Taylor4, and Kristine Johnson4
Abstract - As part of the All Taxa Biodiversity Inventory (ATBI) of Great Smoky Mountains
National Park (GRSM), select site parameters associated within upland sites were measured
within variable sized pockets of stressed and dead Rhododendron maximum (Great Rhododendron).
During the last 20 years, Great Rhododendron, an important shrub in the southern
Appalachian Mountains, has been dying in small to larger areas from an unknown cause.
With increased visibility of dieback, the study (2006–2009) was conducted at 10 sites in
GRSM and 1 in Nantahala National Forest (NNF). Eleven nematode species were identified,
with no specific trends across locations or by plot treatments in frequencies of occurrences.
Exceptions were Criconemella xenoplax, which was found at all 11 locations and was generally
significantly greater in healthy or control than dieback plots, whereas Helicotylenchus
sp. was more frequent in dieback plots. Meloidogyne spp., known parasitic nematodes of
woody plants and agricultural crops, occurred in over 50% of the locations; Hoploliamus sp.
occurred at 40%; and Belomolaimus sp., which are very destructive to root systems, were
found at low levels at the NNF site. Also, Heterodera sp. occurred in control and dieback
plots in 10 of the locations. Dieback ratings were not significantly correlated to stem diameter;
although the finding was not statistically significant, six of seven sites with dieback
plots had numerically greater-sized stems than in the controls. No other parameters such as
number of clonal units, site aspect, percent slope, or elevation showed any trends at both
sites. Nutrient data did not indicate any specific relationships to plot damage or health. This
study provides the first comprehensive reporting of nematode species that occur in Great
Rhododendron and associated riparian and upland sites. With concerns of global climate
impacts, this research provided additional baseline data for the ATBI of GRSM and NNF.
Introduction
Over the last 15 years, the All Taxa Biodiversity Inventory (ATBI) conducted by
Discover Life in America (DLIA) has provided pertinent data to the Great Smoky
Mountains National Park (GRSM) personnel for management considerations and
monitoring of forest health problems (Baird et al. 2007, 2009). Baseline data of
fungal populations have been determined within select forest tree species, such
as Tsuga canadensis (L.) Carr. (Eastern Hemlock), that are being impacted by exotic
pests. With the uncertainty of a causal agent(s) of an emerging rhododendron
1Department of BCH-EPP, 2Plant and Soil Sciences, Mississippi State University, Mississippi
State, MS 39762. 3Arkansas Agricultural Experiment Station, AFLS 212, University
of Arkansas, Fayetteville, AR 72701. 4Vegetation Unit, Great Smoky Mountains National
Park, 107 Park Headquarters, Gatlinburg, TN 37738. *Corresponding author - Richard
Baird, rbaird@plantpath.msstate.edu.
R. Baird, A. Wood-Jones, J. Varco, C. Watson, W. Starrett, G. Taylor, and K. Johnson
2013 Southeastern Naturalist Vol. 12, No. 4
704
decline in the GRSM and surrounding national forests, a survey on potential
parasitic organisms and site features within the affected upland areas may provide
insight as to the cause of the problem. Since no known microbes have been identified
as causal agents in preliminary unpublished studies (Kelly Ivor, North Carolina
State University, Raleigh, NC, pers. comm.; Steve Oak, USDA Forest Service,
Asheville, NC, pers. comm.), parasitic nematode species might be involved, but
little to no data exists to confirm this hypothesis.
In terms of forest ecology and management, Rhododendron maximum L.(Great
Rhododendron) is an important subcanopy shrub to small tree in the GRSM and is
considered a keystone species for the Appalachian forests (Nilsen et al. 1999, Yeakley
et al. 1994). Great Rhododendron has been shown to play a role in soil horizon
and profile formation due to release of degradation products from its slowly decomposing
recalcitrant litter (Boettcher and Kalisz 1990). At the turn of the century and
early in the history of the GRSM, many forest disturbances occurred, such as loss of
the Castanea dentata (Marsh.) Borkh. (American Chestnut), creating successional
changes that had an impact on forest conditions (Rivers et al. 1999) and allowing
Great Rhododendron to extend its influences well beyond the niche in which it
originally occupied.
In the late 1980s, areas of dead Great Rhododendron were reported at upland
sites in the GRSM, and loss of this species could endanger other organisms (Ownley
1993). Rhododendron spp. habitats are of importance for numerous organisms
including a rare orchid, Listera smallii Weigand (Appalachian Twayblade; Radford
et al. 1968, Robinette 1974, Vandermast and Van Lear 2002). Research and study
about the ecological importance of Great Rhododendron and its possible competitive
inhibition effects has been conducted through a number of experiments, further
attesting to the major role Great Rhododendron has in the southern Appalachians
(Baker and Van Lear 1998, Clinton 2003, Clinton et al. 1994, McGee and Smith
1967, Monk et al. 1985, Nilsen et al. 1999, Smith 1963, Yeakley et al. 1994).
Dieback, decline, or loss of this Ericaceae member is of concern. Over the last 25
years, Park Service personnel observed Great Rhododendron plants dying or dead
throughout the park, and damaged areas in 14 stands were large enough to elicit
concern over the survival of Great Rhododendron within the park (G. Taylor, pers.
observ.).
Rhododendron dieback was first studied in the early 1990s, with evaluations of
aboveground parameters at Cerulean Knob area of GRSM (Ownley 1993, 1994).
That study attempted to isolate causal agent(s) from aboveground plant tissues, but
the report was negative for any pathogens. The report addressed the need for continued
soil and root analysis before any further understanding of the problem could
be determined.
Following the initial study, Great Rhododendron dieback continued to be a
problem throughout GRSM and in other diverse areas such as the Nantahala National
Forest (NNF), but its causal factor(s) remained undetermined. The National
Park Service (NPS) reported in 1999 and 2000 that the problem possibly was attributed
to a fungal agent (e.g., Botryosphaeria sp.) (NPS 2004a, b). Based on his
previous study, Ownley (1994) observed that in most cases the entire plant was
705
R. Baird, A. Wood-Jones, J. Varco, C. Watson, W. Starrett, G. Taylor, and K. Johnson
2013 Southeastern Naturalist Vol. 12, No. 4
stressed or in decline with no known causes identified. This problem could be better
defined as a decline in which a gradual reduction of species health is observed
in the aboveground characteristics of the plants (Holliday 2001).
Species of rhododendrons are affected with many stressors, and most have only
been studied from a horticultural perspective. Abiotic stresses such as mineral
deficiencies or excesses, unnatural pH levels, exaggerated environmental conditions,
and air pollution can enhance plant stress, especially on marginal forest sites.
Similarly, bacteria, viruses, nematodes, algae, and fungi, can be causal pathogenic
agents, while others are incidental (Coyier and Roane 1988). These stresses, both
abiotic and biotic, interact with the host plant, affecting possible disease resistance.
Mineral nutrient excesses and deficiencies can impact survivability of Great
Rhododendron (Fink 1999). Stunted growth, chlorosis, discoloration, leaf spots, leaf
loss, and dieback can all be observed due to the levels of specific macro- (N, P, K, Ca,
Mg) and micro- (Fe, B, Mn, Cu, Zn, Mo, Cl) nutrients. In some cases, secondary effects
from biotic factors, such as root diseases and damage to mycorrhizae, reduce
the nutrient uptake ability of the host (Fink 1999). Reduced nutrients have also been
attributed to the cause of biotic infections by allowing parasites to attack stressed
hosts (Agrios 2005). In young rhododendron leaves, a decrease in the facultative
concentration of nitrogen may aid in reducing the incidence of Phytophthora dieback
caused by an organism belonging to Chromalveolata (= Stramenopila) (Benson and
Hoitink 1988). Calcium imbalances affect the meristems and juvenile tissues, which
can result in distortions and decay of the shoots, stems, roots, flowers, and fruits
(Agrios 2005, Fink 1999). Potassium regulates plant turgor and viability, while magnesium
is essential for photosynthesis and, if deficient, may alter the root-to-shoot
ratio by a reduction of root mass (Fink 1999). Magnesium deficiency has also been
linked with forest decline (Fink 1999). Therefore, it is important to evaluate soil nutrients
to ensure a viable diagnosis based on possible abiotic causes.
The occurrence of air pollution and its impact on plant physiology and induced
disease has been observed for over a century (Agrios 2005). Rain becomes acidified,
changing the pH of soils, and certain nutrients can be affected when too much
nitrogen is deposited into the soil. Soil pH level is important for rhododendrons,
with the best growth occurring in a more acidic soil. An increase in pH towards a
more basic soil may allow for the establishment of Phytophthora and the fungus
Phymatotrichum spp. (Benson and Hoitink 1988). Although species of Rhododendron
are usually very tolerant to pH changes, extremes of these conditions can
impinge on their health, causing the plants to become susceptible to other diseases
(Agrios 2005, Sinclair et al. 1993). It has been reported that Great Rhododendron
cannot assimilate iron in an alkaline soil, which ultimately leads to chlorosis and
loss of vital functions; thus, the plants would need an acidic soil with a pH between
4.5 and 5.0 (Bowers 1960). If plenty of organic material is available in the soil, a
pH of 5.5 may be suitable.
Rhododendron dieback or decline is becoming widespread in the GRSM and
surrounding areas. The problem is becoming more evident, with an increasing
number of reported sightings. It is unknown to what extent the impact of the loss of
rhododendron outside the riparian zones will be since all dieback sites are primarily
R. Baird, A. Wood-Jones, J. Varco, C. Watson, W. Starrett, G. Taylor, and K. Johnson
2013 Southeastern Naturalist Vol. 12, No. 4
706
located in upland areas, and no data was available on replacement species. In addition,
rhizosphere data on upland sites within GRSM and NNF on associated root
organisms were provided as All Taxa Biodiversity Inventory (ATBI) baseline information.
Thus, a holistic research approach was conducted to determine if select
abiotic and or biotic factors are associated with this problem. The purpose of this
study was to evaluate dieback areas to 1) define and further characterize rhododendron
dieback symptoms, 2) evaluate site factors including aspect, slope, pH, and
soil fertility, 3) quantify nematode diversity/densities for comparisons with disease
ratings and to contribute the resulting species lists to the ATBI, and 4) collect rhododendron
plant measurements and growth data to determine plant growth variability
between dieback and control plots.
Materials and Methods
Rhododendron decline site evaluations
We studied ten areas of rhododendron dieback within GRSM and one in NNF
(Fig. 1); all were surveyed between May and August 2006, and we took soil samples
Figure 1. Locations of ten Rhododendron maximum (Great Rhododendron) dieback sites in
Great Smoky Mountains National Park (1 = Cerulean Knob, 2=Russell Field, 3 = Laurel
Falls, 4 = Huskey Gap, 5 = Nolan Divide, 6 = Deep Low Gap, 7 = Brushy Mt., 8 = Greenbrier
Pinnacle, 9 = Newton Bald, 10 = Gabe Mt.) and one site in NantahalaNational Forest
(11 = Albert Mountain).
707
R. Baird, A. Wood-Jones, J. Varco, C. Watson, W. Starrett, G. Taylor, and K. Johnson
2013 Southeastern Naturalist Vol. 12, No. 4
in July and August 2007. Mapped soil series and textures, and dominant forest
types are listed for each survey area (Table 1). All soils ranged from shallow to very
deep and all were classified as well drained, except Craggey, which was somewhat
excessively drained. Textures ranged from loam to sandy loam to sandy clay loam.
All soils formed from colluvium, except the Santeetlah and Spivey series, which
formed in place from residuum parent material. The forest ecotypes ranged from
cove hardwoods at three locations to a Montane cove type. Other sites were mixed
or oak forests and occurred in upland sites. Albert Mountain had the most visible
rhododendron dieback damage and was located in an upland oak forest type.
At each of the 11 rhododendron dieback sites, an attempt was made to establish
identical-sized control plots for comparison. Within each site, there were two
treatments, dieback and control, with four subplots within each treatment. Control
plots were defined as healthy areas that contained no visible dieback. Only seven
of the locations contained sufficient areas of healthy plants for control plots to be
established near or adjacent to the damaged areas at similar elevations. At each
site, we established one dieback and one control plot, each consisting of a 100-m
× 100-m area.
To collect rhododendron health data, we used a line-transect method to observe
and tally the incidence of dieback in each plot per location. From the center of
the plot, we established four 57.7-m transects starting 3 m from the midpoint and
ending 10 m from each corner (O’Neill et al. 2005). We tallied all plants located
Table 1. Soil and forest types of the rhododendron dieback (DB) and control (CDB) study sites used
during general survey of site parameters associated with their decline in Great Smoky Mountains
National Park and Nantahala National Forest. (See Fig. 1 for map of site locations).
Site Soil type Forest type
Albert Mt. (DBAM, CDBAM) Burton-Craggey sandy loam Upland oak type
(Nantahala N. F.)
Gabe Mt. (DBGM, CDBGM) Ditney Unicoli loam, gravelly loam Cove hardwood type
Nolan Divide (DBND, CDBND) Oconaluftee-Guyot-Cataloochee Northern hardwood
complex; channery loam, loam type
Cerulean Knob (DBCK) Ditney Unicoi-loam, gravelly loam Mixed hardwood,
acid type
Russell Field (DBRF, CDBRF) Breakneck Pullback channery loam, Mesic oak/
sandy loam hardwood type
Laurel Falls (DBLF, CDBLF) Snowbird loam-sandy clay loam Montane cove
hardwood type
Greenbrier Pinnacle (DBGP, Breakneck Pullback channery Cove hardwood type
CDBGP) loan, sandy loan
Husky Gap (DBHG) Soco-Stecoah channery loam, sandy loam Submesic oak/
hardwood type
Newton Bald (DBNB, CDBNB) Soco-Stecoah channery loam, sandy loam Submesic oak/
hardwood type
Deep Low Gap (DBDLG) Soco-Stecoah channery loam, sandy loam Mixed hardwood,
acid type
Brushy Mt. (DBBM) Spivey-Santeetlah loam, boulder sandy Cove hardwood type
loam
R. Baird, A. Wood-Jones, J. Varco, C. Watson, W. Starrett, G. Taylor, and K. Johnson
2013 Southeastern Naturalist Vol. 12, No. 4
708
within 2 m on either side of the transect. Each Great Rhododendron plant >3 cm
diameter at breast height (dbh) at 50 cm above the ground was evaluated as per
Ownley (1994). A qualitative ranking for each plant was based on leaf whorls per
branch (WH), percentage of leaves with chlorosis (CH), and percentage of dead
twigs and branches (TW) (Table 2). We then analyzed these values using the rhododendron
dieback rating formula (Ownley 1994):
R_Calc = (0.85 / 3)(WH) + (0.85 / 3)(CH) + (0.85 / 3)(TW)
Ratings (R_Calc) can range from 1 to 7, with 1 defined as a heal thy plant and 6
or 7 as dead before or after leaf abscission, respectively. We used the R_Calc value
to determine the severity of dieback for each of the 11 sites including control plots.
We also measured dbh for each plant along each transect.
General site evaluations
Within each site, there were two treatments, dieback and control, with four
subplots within each treatment. In addition to the rating system of the plants, a
general site evaluation was conducted from May through August 2007 on each
of the 11 locations, including aspect, slope, soil pH, soil nutrients, and nematode
levels. We measured and obtained site factors including aspect and slope in each
of four 16 × 16 m subplots nested within each 100-m × 100-m plot using sampling
methods previously described (O’Neill et al. 2005). At the center of the plot, one
subplot was surrounded by three subplots offset from each other and oriented at
120° angles at a distance of 37 m from the centers. Refer to O’Neill et al. (2005)
for a plot diagram.
Within each large dieback and control subplot, we calculated slope using the
percent scale of a Suunto clinometer (Suunto, Carlsbad, CA). Measurements were
done 5 m uphill and 5 m downhill from each subplot center. We averaged percent
slope measurements to obtain slope in each subplot.
We measured litter depth and depth to bedrock within each of the four subplots
of all plots established for the study. Inserting a 1.0-m steel rod into the soil to the
Table 2. Three criteria used to estimate rhododendron dieback, and the values assigned to different
categories.
Criteria Value Criteria Value
Number of whorls (TH) % leaf chlorosis (CH)
≥4 whorls 1 0.0 % 1
3–4 whorls 3 0.0–10.0% 2
2–3 whorls 4 10.0–25% 3
1–2 whorls 5 >25% 5
Recent deadA 6
DeadB 7 Proportion of dead twigs and branches (TW)
<5% 1
5–20.0% 4
>20.0% 5
ARecent dead refers to a dead plant prior to leaf abscission.
BDead plants with no leaves attached.
709
R. Baird, A. Wood-Jones, J. Varco, C. Watson, W. Starrett, G. Taylor, and K. Johnson
2013 Southeastern Naturalist Vol. 12, No. 4
top of the mineral soil layer (A horizon), we measured litter depths from the length
of the steel rod’s penetration. We measured aspect using a Silva Ranger 515.
We collected soil samples for soil-testing analysis and nematode surveys in July
and August 2007. Prior to soil sampling, we removed forest litter (O horizon) to bare
soil (A horizon) from each of the sampling points within each general site factor subplot.
We collected a total of eight soil samples from each subplot per location (four
each for soil testing analysis and nematode detection). To obtain each sample, we
randomly collected nine sub-samples (15 to 20 cm depth starting at O horizon) per
subplot, pooled them into a 19-L polypropylene bucket, and thoroughly mixed them
to ensure a homogenized sample. A volume of 500 ml was collected from the homogenized
samples, placed in a 946 ml Ziploc® plastic bag, and stored until submission
to the Extension Soil Testing Laboratory, Mississippi State University (MSU) for
soil test analysis (Mississippi State University 2004, 2005). Soils were stored in a
cooled ice chest (0 to 10 °C), transferred to refrigeration (4 °C), and prepared for
overnight shipping to the respective diagnostic laboratory units at MSU. Note that we
disinfected sampling tools and bucket using 0.525% (w/v) aqueous NaOCl between
each sampling of the four to eight subplots per location. We placed an additional
homogenized sample of 500 ml from each subplot into a nematode soil sample bag
obtained from the Disease Diagnostic Laboratory, Entomology and Plant Pathology
Department, MSU. Methods for nematode extraction follow those by Baker (1978).
In addition, North Carolina-style semi-automatic elutriator and sugar centrifugation
was performed to isolate the juvenile, vermiform, and cyst stages of nematodes from
the soil (C. Balbalian, Diagnotic Lab., MSU, pers comm.). Number of cysts were determined
by placing 240 g of soil onto a Baermann funnel (Baermann funnel method)
for five days, and numbers of larvae were counted after hatching (Baker 1978). Routine
soil test analysis included pH (1:2 soil:d.i. H2O); soil organic matter; extractable
P, Ca, Mg, K, and Na; percent organic material (OM); and effective cation exchange
capacity (ECEC; sum of cations extracted) (Cox 2001).
Statistical analysis
We analyzed data as a series of combined experiments (combined across
sites) using the GLM procedure of SAS (SAS Institute, Cary, NC), and separated
means using Fisher’s protected least significant difference (LSD). Data
were pooled and subjected to stepwise multiple regression analysis to evaluate
the effect of the measured variables on dieback rating (R_Calc). We pooled all
data and conducted regression analysis to verify and/or modify the existing rhododendron
dieback rating formula. Please note that since 7 of the 11 sites had
control plots due to availability, some analyses were conducted between plots
from just those locations.
Results
We identified eleven species of nematodes from soil samples collected during
the general survey (Table 3). In addition, we recorded cysts of unknown
species, but those numbers were low and not common across locations. Of the
R. Baird, A. Wood-Jones, J. Varco, C. Watson, W. Starrett, G. Taylor, and K. Johnson
2013 Southeastern Naturalist Vol. 12, No. 4
710
Table 3. Percent frequenciesA (and total numbers) of nematode speciesB present in dieback and control Rhododendron maximum (Great Rhododendron)
plots at ten locations during a general survey of site parameters in the southern Appalachian Mountains. M sp. = Meloidogyne sp., P. sp. = Pratylenchus
sp., He. sp. = Helicotylenchus sp., P. m. = Paratrichodorus minor, X. a. = Xiphinema americanum, C. x. = Criconemella xenoplax, Ho. sp. = Hoplolaimus
sp., Het. sp.= Heterodera sp., T. sp. = Tylenchorhynchus sp., R. r. = Rotylenchulus reniformis, B. sp. = Belonolaimus sp., and Others = unidentified cysts
and larvae.
Location M. sp. P. sp. He. sp. P. m. X. a. C. x. Ho. sp. Het. sp. T. sp. R. r. B. sp. Others
Albert Mt.
Dieback 1.2 (79) 3.5 (223) 7.8 (505) <1.0 ( 32) 0.0 (0) 20.8 (1343) 0.0 (0) 0.0 (0) 0.0 (0) <1.0 (40) 0.0 (0) 0.0 (0)
Control 0.0 (0) 3.2 (212) 2.5 (166) <1.0 (6) 0.0 (0) 60.2 (3887) 0.0 (0) 0.0 (0) 0.0 (0) 0.0 (0) <1.0 (21) 0.0 (0)
Brushy Mt.
Dieback 0.0 (0) 0.0 (0) 38.0 (434) 2.1 (24) 0.0 (0) 32.9 (371) 0.0 (0) 25.8 (292) 0.0 (0) 0.0 (0) 0.0 (0) 0.0 (0)
Cerulean Knob
Dieback 0.0 (0) 6.4 (112) 35.4 (615) 0.0 (0) 0.0 (0) 40.4 (702) 5.5 (95) 12.3 (214) 0.0 (0) 0.0 (0) 0.0 (0) 0.0 (0)
Deep Low Gap
Dieback 85.0 (141) 0.0 (0) 12.4 (206) <1.0 (8) <1.0 (20) 60.0 (993) 0.0 (0) 18.1 (299) 0.0 (0) 0.0 (0) 0.0 (0) <1.0 (20)
Gabe Mt.
Dieback <1.0 (13) 0.0 (0) 18.6 (228) 0.0 (0) 0.0 (0) 12.5 (153) 5.8 (71) 8.6 (105) 0.0 (0) 0.0 (0) 0.0 (0) 0.0 (0)
Control 0.0 (0) 0.0 (0) 3.9 (48) 0.0 (0) 0.0 (0) 19.9 (245) 0.0 (0) 29.6 (363) 0.0 (0) 0.0 (0) 0.0 (0) 0.0 (0)
Greenbrier
Dieback 0.0 (0) 0.0 (0) 26.4 (907) 0.0 (0) 0.0 (0) 1.9 (410) 0.0 (0) <1.0 (8) <1.0 (8) 0.0 (0) 0.0 (0) 0.0 (0)
Control 2.5 (86) 0.0 (0) 31.2 (1071) <1.0 (6) 0.0 (0) 24.3 (835) 0.0 (0) 2.5 (87) <1.0 (16) 0.0 (0) 0.0 (0) 0.0 (0)
Husky Gap
Dieback 0.0 (0) 0.0 (0) 52.3 (631) 0.0 (0) 0.0 (0) 33.4 (403) 0.0 (0) 0.0 (0) 0.0 (0) 0.0 (0) 0.0 (0) 13.0 (157)
711
R. Baird, A. Wood-Jones, J. Varco, C. Watson, W. Starrett, G. Taylor, and K. Johnson
2013 Southeastern Naturalist Vol. 12, No. 4
Table 3, continued.
Location M. sp. P. sp. He. sp. P. m. X. a. C. x. Ho. sp. Het. sp. T. sp. R. r. B. sp. Others
Laurel Falls
Dieback 0.0 (0) 0.0 (0) 6.8 (237) 0.0 (0) 0.0 (0) 10.1 (412) 5.6 (62) 6.0 (84) 0.0 (0) <1.0 (12) 0.0 (0) 0.0 (0)
Control 3.2 (112) 0.0 (0) 48.6 (502) 0.0 (0) 0.0 (0) 15.6 (525) 3.0 (43) <1.0 (29) 0.0 (0) 0.0 (0) 0.0 (0) 0.0 (0)
Newton Bald
Dieback 1.8 (64) 0.0 (0) 39.6 (1426) <1.0 (24) <1.0 (24) 12.9 (464) <1.0 (8) 9.2 (332) 0.0 (0) 0.0 (0) 0.0 (0) 0.0 (0)
Control 0.0 (0) 0.0 (0) 7.4 (268) 0.0 (0) 0.0 (0) 29.1 (1048) 0.0 (0) <1.0 (24) 0.0 (0) 0.0 (0) 0.0 (0) 0.0 (0)
Nolan Divide
Dieback 0.0 (0) 0.0 (0) 23.6 (892) 0.0 (0) 0.0 (0) 14.2 (539) 0.0 (0) 2.3 (89) 0.0 (0) 0.0 (0) 0.0 (0) 9.8 (370)
Control 0.0 (0) 0.0 (0) 10.2 (384) 0.0 (0) <1.0 (8) 33.2 (1253) 0.0 (0) <1.0 (8) 0.0 (0) 0.0 (0) 0.0 (0) 6.2 (236)
Russell Field
Dieback 0.0 (0) 0.0 (0) 41.3 (898) 0.0 (0) 0.0 (0) 9.8 (214) 2.9 (64) <1.0 (8) <1.0 (8) 0.0 (0) 0.0 (0) 0.0 (0)
Control 1.7 (39) 0.0 (0) 22.8 (497) 0.0 (0) 0.0 (0) 9.8 (213) <1.0 (8) 2.5 (87) <1.0 16) 0.0 (0) <1.0 (16) 0.0 (0)
A Percent (%) frequencies are based on mean total number of nematodes calculated across dieback and control Great Rhododendron replicate subplot
samples for each location.
BNine soil core samples were pooled from each replicate of four subplot within each large area plots (100 m × 100 m) per location.
R. Baird, A. Wood-Jones, J. Varco, C. Watson, W. Starrett, G. Taylor, and K. Johnson
2013 Southeastern Naturalist Vol. 12, No. 4
712
total species identified, Criconemella xenoplax Raski (ring), Helicotylenchus sp.
(spiral), and Heterodera sp. (sheath) were found at almost all locations. In addition,
Meloidogyne sp. (root knot) occurred at six of the locations during the survey. For
C. xenoplax, population levels were always greater in the control than dieback subplots.
Helicotylenchus sp. levels were generally greater in the dieback than control
areas, whereas levels varied for Heterodera and Meliodogyne sp. Percent frequencies
of Meloidogyne sp. were also greater in dieback subplots (Table 3).
We compared mean numbers of nematode species among sites and within dieback
and control plots (Table 4). Heterodera sp. had significantly greater mean
population levels from soils collected in the dieback subplots and were almost twice
the mean totals, whereas C. xenoplax had consistently greater numbers in control
plots. For each nematode species, we compared mean totals across sites and within
the dieback and control plots (Table 5). Similar results between treatments were
noted as observed for data located in Table 4.
We rated dieback and control subplots for plant diameter, dieback ratings, and
number of clonal units. All seven survey sites containing dieback and control subplots
had significantly greater dieback ratings in the dieback compared to control
subplots (Table 6). Albert Mountain had dieback ratings of 6.3 in the dieback subplots
compared to 2.3 in the adjacent control subplots. Only at the Laurel Fall site
were diameter measurements in the dieback subplots significantly greater (7.4) than
in control sites (5.2). At Russel Field, mean number of clonal units in the dieback
subplots were significantly greater at Russell Field than contro l areas.
Table 4. Comparison of mean number for each nematode speciesA (balanced mean data) compared between
dieback and control Rhododerndron maximum (Great Rhododendron) subplots at six locations
within Great Smoky Mountains National Park and one in Nanatahala National Forest. LSD = Fisher’s
protected least significant difference (P < 0.05).
Nematode species/type Dieback Control LSD
Meloidogyne root knot 2.6 AB 6.5 A 6.4
Pratylenchus lesion 4.0 A 3.9 A 3.9
Helicotylenchus spiral 98.6 A 87.8 A 36.5
Paratrichodorus minor stubby root 1.1 A 0.0.4 A 1.3
Xiphinema americanum dagger 0.1 A 0.0.0 A 0.2
Criconemella xenoplax ring 88.2 B 169.5 A 54.2
Hoplolaimus lance 7.7 A 3.3 A 5.1
Heterodera sheath 21.1 A 10.0.5 B 9.8
Tylenchorhynchus stunt 0.1 A 0.3 A 0.2
Rotylenchulus reniformis reniform 0.0 A 0.0 A 0.0
Belonolaimus sting 0.0.A 0.3 A 0.4
Cysts spp.C (other) 6.2 A 4.3 A 2.1
AAt the seven locations, each treatment (dieback and control) had nine randomly collected soil samples
from four subplots located within the 100-m × 100-m plots sampled in 2007.
BTreatment means followed by the same letter within nematode species are significantly different
(P < 0.05) according to the LSD.
CTotal cysts were counted and then placed onto a Baermann funnel (Baermann funnel method) for five
days, then the number of hatched larvae were counted, and these larvae believed were believed to
be Heterodera spp.
713
R. Baird, A. Wood-Jones, J. Varco, C. Watson, W. Starrett, G. Taylor, and K. Johnson
2013 Southeastern Naturalist Vol. 12, No. 4
Table 5. Comparison of mean number nematode species between dieback and healthy Rhodendron maximum sites at 10 locations within Great Smoky
Mountains and 1 in Nantahala National Forest. Me. = Meloidogyne root knot, Pr. = Pratylenchus lesion, Hel. = Helicotylenchus spiral, P. m. = Paratrichodorus
minor stubby root, X. a. = Xiphinema americanum dagger, C. x. = Criconemella xenoplax ring, Ho. = Hoplolaimus lance, Het. = Heterodera
sheath, Ty. = Tylenchorhynch stunt, R. r. = Rotylenchulus reniformis, Be. = Belonolaimu sting, and Others = Cysts spp.C.
Nematode species (balanced mean data)
LocationsA Me. Pr. Hel. P. m. X. a. C. x. Ho. Het. Ty. R. r. Be. Others
Albert Mt.
Dieback 4.0 BCB 12.0 B 25.3 D–F 1.6 AB 0.0 B 67.2 CD 0.0 C 0.0 C 0.0 C 0.0 C 0.0 C 0.0 D
Control 0.0 C 11.8 B 8.3 E 0.0.4 B 0.0 B 194.4 A–D 0.0 C 0.0 C 0.0 C 0.0 C <1.0 C 0.0 D
Brushy Mt.
Dieback 0.0 C 0.0 B 10.08.5 C–E 6.0 A 0.0 B 66.8 B–D 0.0 C 73.0 A 0.0 C 0.0 C 0.0 C 2.0 D
Caerulean Knob
Dieback 0.0 A 28.0 A 153.8 B–D 0.0 B 0.0 B 175.5 A–D 23.8 A 53.5 AB 0.0 C 0.0 C 0.0 C 0.0 D
Deep Low Gap
Dieback 35.3 A 0.0 B 51.5 D–E 2.0 AB 2.0 A 248.3 A–C 0.0 C 74.8 A 0.0 C 0.0 0.0 B 0.0 D
Gabe Mt.
Dieback 3.3 BC 0.0 B 57.0 DE 0.0 B 0.0 B 38.3 D 18.3 AB 26.3 BC 0.0 C 0.0 0.0 B 0.0 D
Control 0.0 C 0.0 B 12.0 E 0.0 B 0.0 B 61.3 D 0.0 C 90.0.8 A 0.0 C 0.0 0.0 B 0.0 D
Greenbrier
Dieback 0.0 C 0.0 B 226.8 A–C 2.0 AB 0.0 B 10.02.5 B–D 0.0 C 2.0 C 2.0 B 0.0 0.0 B 0.0 D
Control 21.5 AB 0.0 B 267.8 A–C 2.0 AB 0.0 B 20.08.8 AD 0.0 C 21.5 BC 4.0 A 0.0 0.0 B 0.0 D
Huskey Gap
Dieback 0.0 C 0.0 B 157.8 B–D 4.0 AB 0.0 B 65.3 CD 0.0 C 6.0 C 0.0 C 0.0 0.0 B 39.3 C
Laurel Falls
Dieback 0.0 C 0.0 B 52.9 DE 0.0 B 0.0 B 10.03.4 B–D 16.3 A–C 24.5 BC 0.0 C 0.0 C 0.0 B 0.0 D
Control 13.4 BC 0.0 B 131.7 B–E 0.0 B 0.0 B 134.4 A–D 9.6 A-C 7.5 C 0.0 C 0.0 C 0.0 B 0.0 D
R. Baird, A. Wood-Jones, J. Varco, C. Watson, W. Starrett, G. Taylor, and K. Johnson
2013 Southeastern Naturalist Vol. 12, No. 4
714
Table 5, continued.
Nematode species (balanced mean data)
LocationsA Me. Pr. Hel. P. m. X. a. C. x. Ho. Het. Ty. R. r. Be. Others
Newton Bald
Dieback 16.0 A–C 0.0 B 356.5 A 6.0 A 2.0 A 116.0 B–D 0.0 C 83.0 A 0.0 C 0.0 C 0.0 B 0.0 D
Control 0.0 C 0.0 B 67.0 DE 0.0 B 0.0 B 262.0 AB 0.0 C 6.0 C 0.0 C 0.0 C 0.0 B 0.0 D
Nolan Divide
Dieback 0.0 C 0.0 B 223.0 A–C 0.0 B 0.0 B 159.8 A–D 0.0 C 21.8 BC 0.0 C 0.0 C 0.0 B 59.0 B
Control 0.0 C 0.0 B 146.2 B–E 2.0 AB 0.0 B 313.3 A 0.0 C 2.0 C 0.0 C 0.0 C 0.0 B 92.5 A
Russell Field
Dieback 9.8 BC 0.0 B 224.5 A–C 0.0 B 0.0 B 53.5 D 16.0 A–C 60.0.6 AB 0.0 C 0.0 C 0.0 B 0.0 D
Control 0.0 C 0.0 B 124.3 C–E 0.0 B 0.0 B 53.3 D 2.0 BC 0.0 C 0.0 C 0.0 C 4.0 A 6.0 D
LSD 21 12.9 14 4.9 1.1 184.2 17.8 42.7 1.8 0.0 1.6 18.6
A Locations consisted of 100- × 100-m plots subdivided into four subplots where nine core samples were randomly taken per subplot and pooled in 2007.
BTreatment means followed by the same letter within nematode spec ies are significantly different (P < 0.5) according to the LSD.
CTotal cysts were counted and then placed onto a Baermann funnel (Baermann funnel method) for five days, then counted the number of larvae that
hatched. All hatched larve were then counted as Heterodera spp.
715
R. Baird, A. Wood-Jones, J. Varco, C. Watson, W. Starrett, G. Taylor, and K. Johnson
2013 Southeastern Naturalist Vol. 12, No. 4
Significant differences in aspect, soil depth, and slope occurred, but no trends
were observed relative to dieback and control plots (Table 7). Significant differences
occurred within locations and treatments (i.e., dieback and control), but no consistent
trends occurred. Aspect was generally uniform among sites except at Albert Mountain,
where dieback was greater than at any other locations. At this location, mean
slope was minimal at 13.6% and mean soil depth was second greatest at 111.8 cm.
Elevation data between dieback and control plots were significantly different across
the survey sites (Table 7). Differences were noted between the locations, with Albert
Mountain having the highest elevations and dieback levels (data not shown). For the
other sites, there was no relationship between dieback levels and elevations.
Table 6. Mean numberA comparisons of select Rhododendron maximum (Great Rhododendron) site
parameters at seven locations within dieback and control plots.
Diameter Dieback R Number of
Treatments (cm)B value ratingC clonesD AspectE Depth (cm) Slope
Albert Mt.
Dieback 8.4 AA 6.3 A 2.6 A 2.0 A 114.7 A 18.4 A
Control 7.1 A 2.3 B 3.2 A 1.6 A 18.9 A 8.8 B
LSD 2.92 0.72 0.91 0.78 16.12 5.0
Greenbrier
Dieback 7.0 A 5.0 A 5.1 A 6.0 A 98.2 A 26.7 A
Control 6.1 A 2.2 B 2.5 B 6.1 A 104.1 B 25.5 A
LSD 5.0 3.75 0.71 0.00 35.40 2.44
Newton Bald
Dieback 8.3 A 6.3 A 2.6 A 4.8 A 95.6 A 31.5 A
Control 7.4 A 5.2 B 3.5 A 4.7 A 98.1 B 32.3 A
LSD 1.85 0.90 1.40 1.26 4.61 3.71
Russell Field
Dieback 5.6 A 4.7 A 4.8 A 5.3 A 95.4 A 16.7 A
Control 3.7 A 1.5 B 1.5 B 4.0 B 122.0 A 11.1 B
LSD 5.80 2.11 1.93 1.16 27.71 4.83
Gabe Mt.
Dieback 8.0 AA 5.3 A 5.0 A 2.7 B 122.0 A 15.0 B
Control 10.0.8 A 2.1 B 2.1 A 6.8 A 114.6 B 21.5 A
LSD 3.20 1.30 1.40 1.25 17.10 2.64
Laurel Falls
Dieback . 7.4 A 5.2 A 3.5 A 5.5 A 97.5 A 27.3 A
Control 5.2 A 2.7 B 3.0 A 5.7 A 104.5 A 26.2 A
LSD 1.22 0.94 1.35 2.45 15.99 7.47
Nolan Divide
Dieback 7.1 A 2.7 A 3.2 A 5.1 A 93.2 A 19.9 A
Control 5.7 A 2.3 A 3.0 A 3.5 B 10.06.6 A 10.0.5 A
LSD 2.40 0.79 0.95 1.10 36.67 5.00
ATreatment means followed by the same letter within locations are significantly different (P < 0.05)
according to the LSD.
BDiameter of trees refers to measurements taken as dbh or breast height.
CDieback ratings include ratings number of whorls (WH), percent leaf clorosis (CH; which included
dead trees), and number of dead twigs on branches (TW).
DNumbers of clones refer to mean number that occurred within each subplot.
ENorth = 1, northwest = 2, northeast = 3, south = 4, southwest = 5, southeast = 6, east = 7, and west = 8.
R. Baird, A. Wood-Jones, J. Varco, C. Watson, W. Starrett, G. Taylor, and K. Johnson
2013 Southeastern Naturalist Vol. 12, No. 4
716
Table 7. Comparison of mean locations parameters between dieback and or control Rhododendron
maximum (Great Rhododendron) sites at 10 locations within Great Smoky Mountains National Park
and 1 in Nantahala National Forest.
Site factorsA
Locations Aspect Soil depth (cm) Slope (%) Elevation (meters)
Albert Mt.
Dieback 2.0 HIB 114.7 AB 18.8 E–G 1541.4 A
Control 1.6 I 108.9 A–C 8.8 H 1545.7 A
Brushy Mt.
Dieback 6.8 A 105.6 A–C 23.9 CD 1033.3 FG
Caerulean Knob
Dieback 5.4 B–D 103.4 A–C 20.9 DE 1029.6 FG
Deep Low Gap
Dieback 4.7 ED 122.0 A 11.2 H 1031.4 FG
Gabe Mt.
Dieback 2.7 GH 122.0 A 15.7 G 977.8 H
Control 6.8 A 114.6 AB 21.5 DE 971.7 H
Greenbrier
Dieback 6.0 AB 98.2 BC 26.7 C 1059.5 F
Control 6.0 AB 104.1 A–C 25.5 C 1015.0 G
Huskey Gap
Dieback 5.0 CD 103.0 A–C 30.3 AB 1191.8 D
Laurel Falls
Dieback 5.5 B–D 97.5 BC 27.3 BC 952.4 HI
Control 5.8 BC 104.5 A–C 26.2 C 940.5 I
Newton Bald
Dieback 4.8 DE 94.3 C 31.5 A 1141.8 E
Control 4.7 DE 98.6 BC 32.3 A 1048.8 FG
Nolan Divide
Dieback 5.1 CD 93.2 C 19.9 EF 1411.2 B
Control 3.5 FG 106.6 A-C 10.5 EF 1421.8 B
Russell Field
Dieback 5.3 B–D 95.4 BC 16.7 FG 1152.1 E
Control 4.0 EF 122.0 A 11.1 H 1315.8 C
LSD 0.80 20.74 1.97 3.45
All locations
Dieback 4.5 A 104.8 A 22.1 A
Control 4.6. A 108.4 A 20.7 B
LSD 0.34 6.37 1.35
ANorth = 1, northwest = 2, northeast = 3, south = 4, southwest = 5, southeast = 6, east = 7, and west
= 8; depth was determined by replicate readings taken with each subplot per plot; slope is in percent
and was determined within each subplot per plot.
BTreatment means followed by the same letter within site factors are significantly different (P < 0.05)
according to the LSD.
717
R. Baird, A. Wood-Jones, J. Varco, C. Watson, W. Starrett, G. Taylor, and K. Johnson
2013 Southeastern Naturalist Vol. 12, No. 4
In an effort to investigate the possibility of soil factors that may be influencing
the decline, multiple differences in soil test results were found, but most were influenced
by location rather than being classified as a control or dieback site affect
(Table 8). For example, soil test results for phosphorous varied from a low of 24 kg/
ha to a high of 92 kg/ha depending on the site. In terms of crop response, however,
only three locations—DBGP, CDBGP, and DBRF—would be classified as “low” in
available P and potentially responsive to applied P fertilizer. Variability in soil pH
from a low of 3.78 to a high of 4.65 could potentially indicate a stress factor, as the
lower end of the range is extremely acidic even for highly acid-tolerant species. The
four sites with pH values of 4.05 or less were all “dieback” classified, otherwise
there did not appear to be a pH trend effect on rhododendron health. Within-site
comparisons showed that in four out of seven sites the subplot classified as dieback
also had the lower pH for that particular site.
Stepwise regression analysis for dependent variable R_calc (disease ratings) as
affected by soil nutrient levels and nematode densities showed no clear associations
(data not shown). The R2 values ranged from 0.0811 to 0.1252 (P ranged between
<0.001 and 0.017) for models involving OM (organic matter), P, Na, and sting and
root knot nematodes.
Table 8. Selected soil test results within 11 dieback/control sites. P, Ca, Mg, K, and Na all given in
kg/ha. ECEC = effective cation exchange capacity (given in centimoles of charge per kg soil). SOM
= soil organic matter.
Site IDA pHB SOM % P Ca Mg K Na ECEC
DBAM 4.40 6.92 92 9 28 143 93 18
CDBAM 4.62 5.92 73 29 22 134 80 15
DBGM 4.05 5.58 40 9 13 121 80 20
CDBGM 4.15 5.24 47 9 14 151 81 19
DBND 4.30 6.31 62 9 30 221 73 18
CDBND 4.20 7.02 90 9 37 190 78 21
DBCK 4.03 5.73 68 37 21 181 95 21
DBRF 4.25 3.85 24 9 14 123 96 14
CDBRF 4.45 5.20 52 9 11 143 72 16
DBGP 3.90 5.24 31 9 18 128 74 18
CDBGP 4.25 3.94 28 9 9 104 94 12
DBNB 4.65 5.09 43 20 39 228 72 12
CDBNB 4.53 4.73 68 27 45 222 69 12
DBDLG 4.53 3.77 32 9 14 127 85 11
DBLF 4.21 6.57 67 20 22 157 65 20
CDBLF 4.10 8.86 69 53 30 160 65 25
DBBM 3.78 8.85 69 9 50 173 69 29
DBHG 4.15 6.17 59 12 29 209 64 19
LSD(0.05) 0.31 2.14 23 NS NS 42 NS 6
ARefer to Table 1 for actual names of each location.
B1:2 soil:d.i.H2O.
R. Baird, A. Wood-Jones, J. Varco, C. Watson, W. Starrett, G. Taylor, and K. Johnson
2013 Southeastern Naturalist Vol. 12, No. 4
718
Discussion
Rhododendron dieback appears to be widespread throughout the GRSM and surrounding
NNF in pockets of various sizes but never greater than two ha. Within the
riparian zones, Great Rhododendron appears to be expanding, which could partially
be due to alleopathic involvement and acidic soils excluding other species (Baker
and Van Lear 1998). However, in the uplands areas where dieback is prevalent,
Great Rhododendron is being replaced by other woody plant species.
A broad diversity of nematode taxa were found on the upland sites; half were
saprophytic and other plant pathogens from forest and agricultural ecosystems.
Criconemella xeroplax is known to be a parasite of fruit trees such as Prunus and
Juglans spp. (Dreistadt et al. 1994, Ruehle 1973). Also, C. xenoplax was reported
to damage Ilex crenata Thunk. (Japanese Holly; Aycock et al. 1976), but direct
association was never mentioned for rhododendron or any Ericaeae species. Criconemella
xenoplax was reported to decrease tree species growth in association
with microbes such as fungus or fungus-like taxa including Fusarium solani (Mart.)
Sacc. and Pythium irregulare Buis. (Powell et al. 1968).
The nematode Helicotylenchus sp. was common among the 11 dieback locations.
It was previously reported that increased levels of Helicotylenchus dihystera
(Cobb) Sher. and Phytophthora cinnamomi Rands were correlated with increased
disease levels in control studies of nursery tree seedlings (Marx and Davey 1969).
In a previous study in Europe, Helicotylenchus digonicus Perry, an ectoparasitic
species, was commonly associated as a parasite with many hardwoods and a few
conifer tree species (Stollarova 1999). In that study, only nematodes diversity and
densities were determined and no microbial associates were evaluated.
In a concurrent study from two dieback sites, microbes were sequenced and
isolated from roots and soil (Baird et al., in press). However, none of the microbes
listed above were found using sequence data or culturing, except the root pathogen
Ilyonectria radicicola (Gerlach & L. Nilsson) Chaverri & C.G. Salgado, which occurred
at Albert Mountain and Laurel Falls Trail sites during the survey (Baird et
al., in press). This pathogen and select nematode species such as Xiphinema bakeri
Williams are implicated in a disease complex of coniferous tree species (Ruehle
1973). Additional research will be necessary to confirm whether a disease complex
between I. radicicola and a nematode species is responsible for rhododendron decline.
Meloidogyne sp. occurred in the current study and is known to be parasitic
on landscape or woody plants and forest trees (Dreistadt et al. 1994, Ruehle 1973).
However, in this study, occurrence of the nematode was scattered and population
levels were low and probably below the threshold for visible damage to occur.
Even though at six of seven sites stem diameters were numerically greater in
dieback plots than controls shown in Table 6, dieback levels were not significantly
correlated with stem diameter (data not shown). Contrary to these results, rhododendron
plant diameters were significantly greater in dieback plots in a study reported
by Ownley (1994). A preliminary study conducted by USDA/Park Service in 1994
compared dbh of 30 rhododendron plants to core sample age. Plant diameter sizes
did not show a significant trend to greater age (growth rings) of stems from the
719
R. Baird, A. Wood-Jones, J. Varco, C. Watson, W. Starrett, G. Taylor, and K. Johnson
2013 Southeastern Naturalist Vol. 12, No. 4
Cerulean Knob dieback area (G. Taylor, unpubl. data). Based on this small sample
size, this preliminary investigation showed a trend that larger diameter plants with
greater disease levels might not be age related.
Soil pH and other nutrient parameters appear to be factors needing further study.
The observation from the analysis suggests that pH alone is not the determining
factor, as other sites with more acidic pH did not behave in the same manner.
Site characteristics and other soil chemical properties not measured here, such as
exchangeable Al levels, may also play a role in dieback progression. Although extractable
soil Ca2+ levels did not differ, the quantities are extremely low for plant
growth. The impact of low levels of Ca2+ on dieback need further study as well as
the possible effects of varying levels of soluble Al and Mn, which were not determined
in this study.
In conclusion, nematode species diversity and density data obtained from the 11
sites were used to determine their association to rhododendron stands for ATBI database
information and to determine if any other site parameters might be involved
in dieback. Those data were submitted to the USDA/Department of Interior, ATBI
database being collected to catalog all organisms in GRSM. This research is the first
comprehensive study of nematodes from various forest types within the park and
southern Appalachian Mountains. Since several of the nematode species are known
to be parasitic on woody plants, the data has further implications for forest health,
especially when considered in conjuction with climate change parameters and nutrient
depositions that affect rhizosphere organisms and subsequent tree growth.
Concerning dieback, the site factors varied across locations overall, though without
any real trends being noted for the 11 sites. Contrary to these results, nematode
occurrence does indicate possible association with rhododendron decline, but additional
research is needed to confirm these initial findings. Also, it is possible that
a nematode-microbe disease complex may cause rhododendron decline within the
Great Smoky Mountains and vicinity. Previous literature supports the hypothesis
that certain nematode species identified in this study are parasitic on woody plants
as discussed in the introduction. Furthermore a disease complex might be involved,
especially since many of the areas where decline occurs (e.g., ridge tops) are not
within areas of optimal growing condition for rhododendron. Another study that
was conducted simultaneously during the current investigation included sampling
for microbes (Baird et al., in press). Ilyonectria radicicola, a known pathogen of
tree roots, was found at two dieback locations in the southern Appalachian Mountains.
Also the low levels of Ca2+ might predispose rhododendron plants to attack
by biotic pests. Because these data indicate certain trends, additional soil-root sampling
for nematodes, microbial pathogens, and select nutrients is needed to confirm
the previous results across many diverse locations and elevations. We recommend
that greenhouse studies confirm the initial field observations.
Acknowledgments
Appreciation is extended to the Great Smoky Mountains Conservation Association
for a Carlos C. Campbell Memorial Fellowship Award in 2007. In addition, financial
R. Baird, A. Wood-Jones, J. Varco, C. Watson, W. Starrett, G. Taylor, and K. Johnson
2013 Southeastern Naturalist Vol. 12, No. 4
720
support of the project was provided by American Rhododendron Society in 2007. Also,
a thank you is extended to Highlands Biological Station for financial support as Grants-
In-Aid during 2007 and 2008. Logistical and technical support of the project provided
by Department of Interior, Park Service, GRSM, is appreciated. We thank David Pratt,
Botany Field Station, University of Tennessee, for providing the laboratory and housing
facilities necessary to support the research. Finally, are grateful to Mississippi State University
for use of laboratory facilities and supplies not covered by grants. This paper is
MAFES publication number 12186.
Literature Cited
Agrios, G.N. 2005. Plant Pathology. 5th Edition. Elsevier Academic Press, Boston, MA.
922 pp.
Aycock, R., K.R. Barker, and D.M. Benson. 1976. Susceptibility of Japanese Holly to
Criconemoides xenoplax, Tylenchorhynchus claytoni, and certain other plant-parasitic
nematodes. Journal of Nematology 8:26–31.
Baird, R.E., C.E. Watson, and S. Woolfolk. 2007. Microfungi from bark of healthy and
damaged American Beech, Fraser Fir, and Eastern Hemlock forests during an all taxa
biodiversity inventory (ATBI) in Great Smoky Mountains National Park. Southeastern
Naturalist 6:67–82.
Baird, R.E., S. Woolfolk, and C.E. Watson. 2009. Microfungi of forest litter of healthy
American Beech, Fraser Fir, and Eastern Hemlock stands from forests in Great Smoky
Mountains National Park. Southeastern Naturalist 8:609–630.
Baird, R., A. Wood-Jones, J. Varco, W. Starrett, G. Taylor, and K. Johnson. In press. Rhododendron
decline in Great Smoky Mountains and surrounding areas: Intensive site study
of biotic and abiotic parameters associated with the decline. Eastern Biologist (In press).
Baker, K.R. 1978. Determining nematode population responses to control agents. Pp.
114–127, In E.I. Zehr (Ed.). Methods for Evaluating Plant Fungicides, Nematicides, and
Bactericides. American Phytopathological Society, St. Paul, MN.
Baker, T.T., and D.H. Van Lear. 1998. Relations between density of rhododendron thickets
and diversity of riparian forests. Forest Ecology Management 109:21–32.
Benson, D.M., and H.A.J. Hoitink.1988. Phytophthora dieback. Pp. 12–15, In D.L. Coyier
and M.K. Roane (Eds.). Compendium of Rhododendron and Azalea Diseases. APS
Press, St. Paul, MN.
Boettcher, S.E., and P.J. Kalisz. 1990. Single-tree influence on soil properties in the mountains
of eastern Kentucky. Ecology 71:1365–1372.
Bowers, C.G. 1960. Rhododendrons and Azaleas. 2nd Edition. Macmillian, New York, NY.
525 pp.
Clinton, B.D. 2003. Light, temperature, and soil moisture responses to elevation, evergreen
understory, and small canopy gaps in the southern Appalachians. Forest Ecology Management
186:243–255.
Clinton, B.D., L.R. Boring, and W.T. Swank. 1994. Regeneration patterns in canopy gaps
of mixed-oak forests of the southern Appalachians: Influences of topographic position
and evergreen understory. American Midland Naturalist 132:308–319.
Cox, M.S. 2001. The Lacaster Soil Test Method as an Alternative to Mehlich 3 Soil Test
Method. Soil Science 166:484–489.
Coyier, D.L., and M.K. Roane (Eds.). 1988. Compendium of Rhododendron and Azalea
Diseases. APS Press, St. Paul, MN. 65 pp.
721
R. Baird, A. Wood-Jones, J. Varco, C. Watson, W. Starrett, G. Taylor, and K. Johnson
2013 Southeastern Naturalist Vol. 12, No. 4
Dreistadt, S.H., J.K. Clark, and M.L. Flint. 1994. Pests of landscape trees and shrubs. An
Integrated Pest Management Guide. University of California, Division of Natural Resources,
Davis, CA. Publication 3359.
Fink, S. 1999. Pathological and Regenerative Plant Anatomy. Gebrüder Borntraeger, Berlin,
Germany. 1095 pp.
Holliday, P. 2001. A Dictionary of Plant Pathology. 2nd Edition. Cambridge University
Press, Cambridge, UK. 536 pp.
Marx, D.H., and C.B. Davey. 1969. The influence of ectotrophic mycorrhizal fungi on the
resistance of pine roots to pathogenic infections. III. Resistance of aseptically formed
mycorrhizae to infection by Phytophthora cinnamomi. Phytopathology 59:549–558.
McGee, C.E., and R.C. Smith. 1967. Undisturbed rhododendron thickets are not spreading.
Journal of Forestry 65:334–336.
Mississippi State University. 2004. Soil testing for the farmer. Available online at http://
msucares.com/pubs/infosheets/is0346.htm. Accessed 3 November 2005.
Mississippi State University. 2005. Plant disease and nematode diagnostic services.
M-1230. Mississippi State Extension Services, Mississippi State, MS.
Monk, C.D., D.T. McGinty, and F.P. Day, Jr. 1985. The ecological importance of Kalmia
latifolia and Rhododendron maximum in the deciduous forests of the southern Appalachians.
Bulletin Torrey Botanical Club 112:187–193.
National Park Service, US Department of the Interior (NPS). 2004a. Inventory and prototype
monitoring of natural resources in selected national park system units 1998–1999.
Available online at http://www.nature.nps.gov/ publications/TechRpt2000-1/glitzi-03.
htm#P49_8562. Accessed 3 November 2005.
NPS. 2004b. Inventory and Prototype Monitoring of Natural Resources in Selected National
Park System Units 1999–2000. Available online at http://www.nature.nps.gov/
publications/TR2001-1/Glitz-04.htm#P262_38085. Accessed 3 November 2005.
Nilsen, E.T., J.F. Walker, O.K. Miller, S.W. Semones, T.T. Lei, and B.D. Clinton. 1999.
Inhibition of seedling survival under Rhododendron maximum (Ericaceae): Could allelopathy
be a cause? American Journal Botany 86:1597–1605.
O’Neill, K.P., M.C. Amacher, and C.H. Perry. 2005. Soils as an indicator of forest health:
A guide to the collection, analysis, and interpretation of soil indicator data in the forest
inventory and analysis program. North Central Research Station, Forest Service, US
Department of Agriculture, St. Paul, MN. General Technical Report NC-258.
Ownley, B.H. 1993. Investigator’s annual report-Part II. National Park Service, US Department
of the Interior, Gatlinburg, TN. GRSM-N-0860083.
Ownley, B.H. 1994. Biotic and abiotic factors associated with rhododendron dieback in the
Great Smoky Mountains National Park. National Park Services, US Department of the
Interior, Gatlinburg, TN. Sub-Agreement to Cooperative Agreement CA-5460-0-9001.
Powell, W.M., F.F. Hendrix, Jr., and D.H. Marx. 1968. Chemical control of feeder root
necrosis of pecans caused by Pythium species and nematodes. Plant Disease Reporter
52:577–578.
Radford, A.E., H.E. Ahles, and C.R. Bell. 1968. Manual of the Vascular Flora of the Carolinas.
University of North Carolina Press, Chapel Hill, NC. 1 183 pp.
Rivers, C.T., D.H. Van Lear, B.D. Clinton, and T.A. Waldrop. 1999. Community composition
in canopy gaps as influenced by presence or absence of Rhododendron maximum.
Pp. 57–60, In J.D. Haywood (Ed.). Proceedings of the Tenth Biennial Southern Silvicultural
Research Conference; 1999 February 16–18, Shreveport, LA. US Department of
Agriculture, Forest Service, Southern Research Station, Asheville, NC. General Technical
Report SRS-30.
R. Baird, A. Wood-Jones, J. Varco, C. Watson, W. Starrett, G. Taylor, and K. Johnson
2013 Southeastern Naturalist Vol. 12, No. 4
722
Robinette, S.L. 1974. Rhododendron, Rhododendron maximum L. US Department of Agriculture,
West Virginia University, Morgantown, WV. Technical Report 9:113–115.
Ruehle, J.L. 1973. Nematodes and forest trees: Types of damage to tree roots. Annual Review
of Phytopathology 11:99–118.
Sinclair, W.A., H.H. Lyon, and W.T. Johnson. 1993. Diseases of Trees and Shrubs. Comstock
Publishing Associates, Ithaca, NY. 575 pp.
Smith, R. C. 1963. Some aspects of variation in growth and development of Rhododendron
maximum L. in western North Carolina. M.Sc. Thesis. North Carolina State University,
Raleigh, NC.
Stollarova, I. 1999. The occurrence, distribution, and abundance of plant parasitic nematodes
in forest and fruit nurseries of Slovakia. Nematologia Mediterranea 27:47–56.
Vandermast, D.B., and D.H. Van Lear. 2002. Riparian vegetation in the southern Appalachian
mountains following chestnut blight. Forest Ecology Management 155:7–106.
Yeakley, I.A., J.L. Meyer, and W.T. Swank. 1994. Hillslope nutrient flux during near-stream
vegetation removal, I. A multi-scaled modeling design. Water, Air, and Soil Pollution
77:229–246.