Toxicity of Three Dispersants Alone and in Combination
with Crude Oil on Blue Crab Callinectes sapidus Megalopae
Rachel Fern, Kim Withers, Paul Zimba, Tony Wood, and Lee Schoech
Southeastern Naturalist, Volume 14, Issue 4 (2015): G82–G92
Full-text pdf (Accessible only to subscribers.To subscribe click here.)
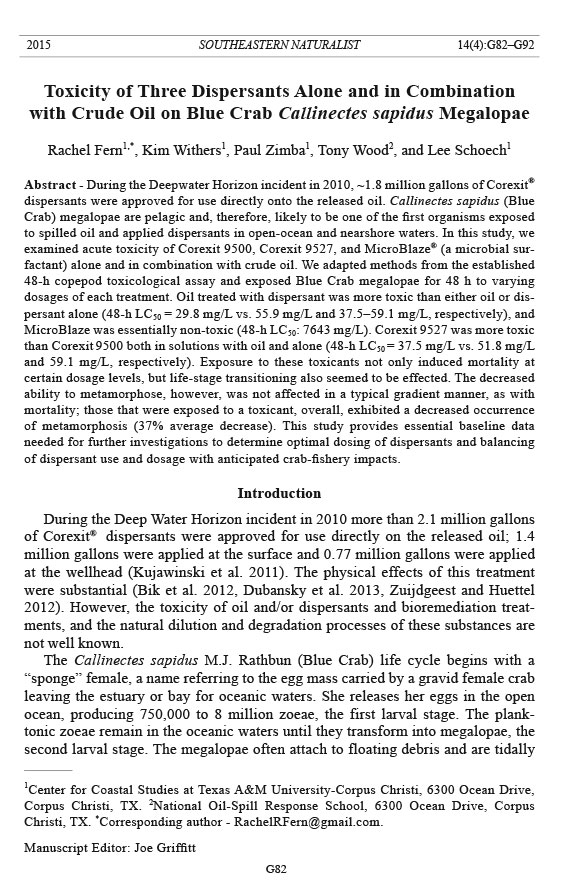
Southeastern Naturalist
R. Fern, K. Withers, P. Zimba, T. Wood, and L. Schoech
2015 Vol. 14, No. 4
G82
2015 SOUTHEASTERN NATURALIST 14(4):G82–G92
Toxicity of Three Dispersants Alone and in Combination
with Crude Oil on Blue Crab Callinectes sapidus Megalopae
Rachel Fern1,*, Kim Withers1, Paul Zimba1, Tony Wood2, and Lee Schoech1
Abstract - During the Deepwater Horizon incident in 2010, ~1.8 million gallons of Corexit®
dispersants were approved for use directly onto the released oil. Callinectes sapidus (Blue
Crab) megalopae are pelagic and, therefore, likely to be one of the first organisms exposed
to spilled oil and applied dispersants in open-ocean and nearshore waters. In this study, we
examined acute toxicity of Corexit 9500, Corexit 9527, and MicroBlaze® (a microbial surfactant)
alone and in combination with crude oil. We adapted methods from the established
48-h copepod toxicological assay and exposed Blue Crab megalopae for 48 h to varying
dosages of each treatment. Oil treated with dispersant was more toxic than either oil or dispersant
alone (48-h LC50 = 29.8 mg/L vs. 55.9 mg/L and 37.5–59.1 mg/L, respectively), and
MicroBlaze was essentially non-toxic (48-h LC50: 7643 mg/L). Corexit 9527 was more toxic
than Corexit 9500 both in solutions with oil and alone (48-h LC50 = 37.5 mg/L vs. 51.8 mg/L
and 59.1 mg/L, respectively). Exposure to these toxicants not only induced mortality at
certain dosage levels, but life-stage transitioning also seemed to be effected. The decreased
ability to metamorphose, however, was not affected in a typical gradient manner, as with
mortality; those that were exposed to a toxicant, overall, exhibited a decreased occurrence
of metamorphosis (37% average decrease). This study provides essential baseline data
needed for further investigations to determine optimal dosing of dispersants and balancing
of dispersant use and dosage with anticipated crab-fishery impac ts.
Introduction
During the Deep Water Horizon incident in 2010 more than 2.1 million gallons
of Corexit® dispersants were approved for use directly on the released oil; 1.4
million gallons were applied at the surface and 0.77 million gallons were applied
at the wellhead (Kujawinski et al. 2011). The physical effects of this treatment
were substantial (Bik et al. 2012, Dubansky et al. 2013, Zuijdgeest and Huettel
2012). However, the toxicity of oil and/or dispersants and bioremediation treatments,
and the natural dilution and degradation processes of these substances are
not well known.
The Callinectes sapidus M.J. Rathbun (Blue Crab) life cycle begins with a
“sponge” female, a name referring to the egg mass carried by a gravid female crab
leaving the estuary or bay for oceanic waters. She releases her eggs in the open
ocean, producing 750,000 to 8 million zoeae, the first larval stage. The planktonic
zoeae remain in the oceanic waters until they transform into megalopae, the
second larval stage. The megalopae often attach to floating debris and are tidally
1Center for Coastal Studies at Texas A&M University-Corpus Christi, 6300 Ocean Drive,
Corpus Christi, TX. 2National Oil-Spill Response School, 6300 Ocean Drive, Corpus
Christi, TX. *Corresponding author - RachelRFern@gmail.com.
Manuscript Editor: Joe Griffitt
Southeastern Naturalist
G83
R. Fern, K. Withers, P. Zimba, T. Wood, and L. Schoech
2015 Vol. 14, No. 4
transported back into estuarine nursery areas where the final transformation into
adulthood occurs (Churchill 1919). Blue Crab larvae are ideal test organisms to
determine toxicities of oil, dispersants, and/or other bioremediation treatment
combinations because the pelagic megalopae are generally planktonic and drift
within the top 2 m of offshore waters (Shanks 1985, Sulkin and Van Heukelem
1982). Larval life-stages of crustaceans are often the organisms most sensitive
to contaminants (Ahsanullah and Arnott 1978, Conner 1972); larvae of Crangon
crangon L. (Sand Shrimp ), Carcinus maenus L. (Green Crab), and Homarus
gammerus L. (European Lobster) were 1 to 3 orders of magnitude more sensitive
to mercury, copper, and zinc than adults. Similarly, Ahsanhullah and Arnott
(1978) found a 9-fold increase in sensitivity to copper, cadmium, and zinc for larval
Paragrapsus quadridentatus (H. Milne Edwards) (Four-toothed Shore Crab)
relative to adults. Early life-stages of Blue Crab are likely to be some of the first
aquatic organisms exposed to oil spills in US waters, and the species’ commercial
significance makes determining toxicity effects of oil and dispersants both ecologically
and economically important. Average US landings of hard-shell Blue
Crabs were 1.6 million pounds during 2006–2010, and the fishery was valued at
more than $180 million in 2011 (NMFS 2012).
Oil dispersants are mixtures of surfactants and hydrocarbon-based solvents
that are used in response to oil spills to break oil into smaller, more dilutable molecular
clusters (Kujawinski et al. 2011). Dispersants reduce the oil’s buoyancy,
keeping it suspended in the water column and preventing oil slicks on the surface
(George-Ares and Clark 2000). Due to the increase in surface-to-volume ratio,
these smaller oil particles are more easily degraded and diluted by microbial communities,
UV rays, and wave action (Atlas 1981, Brakstad 2008, NRC 2005). Two
dispersants were used extensively during the Deepwater Horizon oil spill—Corexit
9527 and Corexit 9500. Corexit 9527 was only applied to the surface of the
spill aerially and by small vessels depending on weather and oil conditions, which
likely resulted in spatially and temporally heterogeneous surface-water concentrations
(Kujawinski et al. 2011). Corexit 9500 was applied both at the surface of
the spill and by a jet placed into the flow of oil and gas emerging from the 150-mdeep
wellhead. However, due to wellhead-operation variability, jet application
was inconsistent, the flow of Corexit 9500 was not constant, and the jet was not
always inserted into the oil and gas stream. It was presumed that when the jet was
properly inserted into the oil and gas flow and Corexit 9500 was being applied,
the dispersant was mixed evenly with the oil as it rose up the water column to the
surface (Kujawinski et al. 2011).
When used appropriately, dispersants can help mitigate coastal impacts in the
early stages of an oil spill (NRC 2005), particularly to habitats such as Rhizphora
spp. (mangroves) and salt marshes (Duke et al. 2000, Getter et al. 1985). Dispersants
alone may not be very toxic, but when added to oil, their toxicity and/or
the toxicity of the oil is compounded (NRC 1989). These chemicals cause large
increases in the number of oil particles dissolved and suspended in the water
column, thus greatly increasing exposure of aquatic organisms (George-Ares and
Southeastern Naturalist
R. Fern, K. Withers, P. Zimba, T. Wood, and L. Schoech
2015 Vol. 14, No. 4
G84
Clark 2000). Severe effects of dispersants on wildlife and microbial communities
have been documented, particularly in coastal ecosystems (Couillard et al. 2005,
Lindstrom and Braddock 2002, UnHyuk and GiMyeong 2009). Peak exposure of
aquatic organisms likely occurs immediately following application of dispersants
and wanes as the dissolved oil particles are diluted. However, repeated or heavy use
of dispersants may damage populations of some pelagic organisms (Couillard et al.
2005, Perkins et al. 1973, Wells 1984). In our study, we determined the toxicity on
Blue Crab megalopae of oil, dispersants, and a non-dispersant bioremediation treatment
alone and in combination, and herein report our observations of the effects of
the various treatments on subsequent metamorphosis.
Methods
Organism collection and lab structure
On several occasions in 2012, we used a 500-mm plankton tow to collect Blue
Crab megalopae directly off the ship channel in Port Aransas, TX. We used all
organisms for experimental trials within 12 h of collection. We based our toxicological
methods on the copepod 48-h toxicity assay (Gorbi et al. 1909). We aerated
the seawater used in the trials for 3 h prior to use, manipulated the salinity with
deionized water to match the salinity of the water from which we collected the
organisms (usually 30–35 PSU), and vacuum-filtered it through a 48-mm filter to
remove organisms and debris from experimental solutions to ensure homogenous
exposure-conditions. In the 48-h copepod toxicity assay, 4–5 copepods were
placed in each 25-mL vial; however, megalopae are larger than copepods, so we
placed only 1 organism in each vial. Before beginning experimental treatments, we
determined megalopae survival under test conditions to ensure oxygen and food
availability would not affect exposure reactions of the megalopae. All megalopae
metamorphosed within 48 hours in the vials and survived for 7 days, more than 3
times the time period for experimental trials. In experimental and control trials,
we isolated the megalopae, introduced 1 into each 25-mL glass vial with 25-mL of
treatment solution for a 48-h exposure period.
Experimental design
For treatments to test dispersant toxicity alone, each trial consisted of 15 individual
megalopae tested at each of 6 concentrations: 0, 20, 50, 80, 110, and
140 mg/L of dispersant. For treatments testing toxicity of oil alone or in an oil
and dispersant combination, trials consisted of 15 individual megalopae tested at
each of 6 concentrations: 0, 15, 37.5, 60, 82.5, and 105 mg/L. Treatments testing
MicroBlaze® (a microbial surfacant) also consisted of 15 individual megalopae
tested at each of 6 concentrations: 0, 2000, 4000, 6000, 8000, and10000 mg/L.
We performed preliminary trials for each treatment across a wide range of concentrations
to determine appropriate concentration intervals for experimental
trials. We performed a total of 4 trials for each treatment with a sample size at
each concentration of 60 megalopae. Within each trial, we examined all concentration
exposures simultaneously (including the control, or 0 mg/L concentration)
Southeastern Naturalist
G85
R. Fern, K. Withers, P. Zimba, T. Wood, and L. Schoech
2015 Vol. 14, No. 4
in order to ensure homogenous testing conditions. Lab conditions remained
constant throughout the 48-h testing periods—72 °F and fluorescent lighting remained
on throughout the period.
In this study, we used Bonny light-crude oil because it was easily accessible
and has an American Petroluem Instutute gravity (API) similar to that of South
Louisiana sweet-crude oil (33.4° and 35.9°, respectively), the oil that was spilled
in the 2010 Macondo blowout. Bonny light-crude sulfur content (0.16%) is lower
than that of South Louisiana sweet crude (0.33%); thus, the former is less corrosive
and less environmentally threatening than the latter, making the results of this study
conservative with regards to their application to the Gulf of Mexico. We dispersed
crude oil with Corexit at the recommended 50:1 ratio. We placed all solutions on
magnetic stir plates, using glass magnetic bullets, and mixed until we observed dissolution
of the oil. MicroBlaze is packaged in concentrated form. We made a 6%
MicroBlaze solution in seawater and mixed experimental concentrations using this
stock solution. We chose a 48-h test period to mimic a realistic oil-spill exposure
because water currents and turbulence generally dilute dispersed oil shortly after
the dispersants are applied. At the termination of each experimental exposure period,
we placed each surviving organism into a new vial containing clean seawater,
and monitored it for an additional 48 hours to determine delayed mortality or delayed
life-stage transitioning.
Reference toxicant and data analysis
Ours is the first study to establish toxicity of these dispersants and dispersed
crude oil on Blue Crab megalopae; thus we used a reference toxicant, sodium dodecyl
sulfate (SDS) to validate methodology and calculate 48-h LC50 values. Our
megalopae SDS-exposure protocol was identical to that of the experimental trials.
This surfactant has been employed in other studies as a reference toxicant and it has
exhibited low variability and high reproducibility (Whiting et al. 1996). Thus, using
SDS as the reference toxicant in this study helped ensure accuracy of our calculated
48-h LC50 values and validate our methodology.
We recorded megalopae mortality and life-stage at the end of the exposure and
post-exposure periods across all treatments. We present our data in a dose-response
curve in order to illustrate relative toxicity of all combinations across increasing
dosages. We made initial examinations of the data using a 2-factor design and
evaluated time x treatment interactions. We conducted our analyses with SAS for
Windows V9. Specifically, we used procedures PROBIT and ANOVA to establish
48-h LC50 values and determine within- and between-group differences, and
Tukey’s HSD to test for significant differences between groups. In all cases, we set
P = 0.05 to indicate significance.
Results
We were able to conduct a 1-way analysis of data because there was no significant
time interaction. All treatments had significant differences among treatment
levels (Table 1).
Southeastern Naturalist
R. Fern, K. Withers, P. Zimba, T. Wood, and L. Schoech
2015 Vol. 14, No. 4
G86
Sodium dodecyl sulfate
We used PROBIT analysis to examine mortality of megalopae on exposure
to SDS to establish a standard 48-h LC50 value. Exposure to SDS resulted in a
consistent mortality gradient across dosage levels, and we derived from the data
a 48-h LC50 SDS value of 5.6 mg/L. This result is lower than the published SDS
48-h LC50 value of 9.8 mg/L for Blue Crab larvae (Whiting et al. 1996). However,
the previously published value was calculated using newly hatched larvae (zoeae),
which may have different physiological requirements. This finding implies that the
megalopae life stage may be more sensitive to toxicants than other stages, most
likely due to the increased physiological demands of metamorphosis that took place
within the vials during exposure (Jacobi and Anger 1985, Mangum et al. 1985).
Corexit 9500, 9527, and dispersed oil
We monitored organisms exposed to Corexit 9500- and Corexit 9500-dispersed
crude oil for acute and delayed mortality (mortality in the 48-h post-exposure
period) and observed none. All control organisms survived and metamorphosed
in the vials during the first 48-hour period. Oil dispersed with Corexit 9527 was
significantly more toxic than any other solution or combination of crude oil and
dispersant (Table 1). The dispersed oil was more toxic than the dispersant alone.
The 48-h LC50 values for Corexit 9500 alone and Corexit 9500-dispersed oil were
59.1 mg/L and 51.8 mg/L, respectively. Although oil dispersed with Corexit 9500
showed a slight tendency to be more toxic than oil or the dispersant alone, the differences
were not statistically significant.
The 48-h LC50 values for Corexit 9527 and Corexit 9527-dispersed oil were 37.5
mg/L and 29.8 mg/L, respectively, which is significantly lower than the Corexit
9500 or Corexit 9500-dispersed oil (Table 1). All megalopae in the control group
survived and metamorphosed in the vials during the 48-h experimental period. We
did not observe delayed mortality in either the control or experimental treatments;
organisms that survived the initial 48-h exposure period ultimately survived the
experiment in its entirety.
Crude oil and MicroBlaze
In order to ensure exposure of the megalopae to oil, trials involving crude oil
alone required the use of a dispersant to adequately suspend the crude oil in a seawater
solution. We used low concentrations of SDS to disperse the oil into water
Table 1. 48-hr LC50 values and ANOVA results comparing dosing level for each treatment compound.
48-hr LC50 Concentration mg/L
Treatment mg/L F value P 140 110 80 50 20 0
C9527 + oil 29.8 263.1 less than 0.0001 - - - - - -
C9500 59.1 408.0 less than 0.0001 - - - - - -
C9527 37.5 772.4 less than 0.0001 - - - - - -
C9500 + oil 51.8 414.4 less than 0.0001 105 83 60 38 15 0
MicroBlaze 7643.0 1065.4 less than 0.0001 10,000 8000 6000 4000 2000 0
Oil 55.9 - - 100 50 25 13 6 0
Southeastern Naturalist
G87
R. Fern, K. Withers, P. Zimba, T. Wood, and L. Schoech
2015 Vol. 14, No. 4
(SDS concentration less than 0.002 mg/L), substantially lower than the SDS no-observedeffect
(NOEC) value of 0.59 mg/L (Whiting et al. 1996). We included an SDS plus
seawater control at this concentration to ensure no toxicity at this dose. The 48-h
LC50 value for crude oil was 55.9 mg/L (Table 1).
MicroBlaze was essentially non-toxic—48-h LC50 = 7643 mg/L (Table 1). We
attempted trials with MicroBlaze and oil combinations, but they resulted in abnormal
dissolution behavior of the crude oil in seawater. When we used MicroBlaze
as the sole dispersant in the solution, crude oil formed compact beads that did not
dissolute until after 48 h. If we had used this solution that had already been exposed
to the microbial surfactant for 48 h prior to the start of the experimental exposure,
it would have yielded biased results because the added time allowed for oxygen and
microbe-aided degradation of the oil. Thus, for the purposes of this study, which
aimed to establish baseline acute toxicity of these chemicals, MicroBlaze-dispersed
oil did not produce comparable or relevant results. However, the effects of this surfactant
on crude oil and (other) dispersant solutions as a bioremediation agent are
currently being investigated.
Life-stage transitioning
Exposure to these toxicants (crude oil and dispersants) not only induced mortality
at certain dosage levels, but life-stage transitioning also seemed to be affected.
The decreased ability to metamorphose, however, was not displayed in a typical
gradient manner, as was mortality; rather, megalopae that were exposed to the
toxicant exhibited a decreased likelihood of metamorphosis (Table 2). Transformation
rates of organisms deceased at the end of the 48-h exposure period differed
from those of organisms that survived the exposure. High transformation-ratios
(alive:deceased) indicated more acute toxic effects of the solution on the organism.
For instance, toxic effects of crude oil alone likely occurred sooner in the exposure
period than those of the Corexit 9500 alone since a great proportion of those in the
latter treatment transformed during exposure.
Our analyses did not account for control organisms because all megalopae in the
control treatments survived and metamorphosed within the initial 48-h exposure
period. Thus, our null hypothesis was that all experimental megalopae would also
Table 2. Metamorphosis frequencies across treatments of organisms that survived or died during the
exposure period. Alive = Organisms that both metamorphosed and survived exposure, Dead = Organisms
that had metamorphosed but died during the exposure period. Total = Cumulative proportion of
organisms that transitioned, dead and alive, during the exposure period..
% Transitioned
Treatment Alive Dead Total
C9500 90.6 100.0 82.3
Oil/C9500 81.3 88.0 77.5
C9527 86.7 95.0 88.0
Oil/C9527 5.3 20.0 1.7
Oil 32.0 46.0 14.0
MicroBlaze 81.3 78.0 93.0
Southeastern Naturalist
R. Fern, K. Withers, P. Zimba, T. Wood, and L. Schoech
2015 Vol. 14, No. 4
G88
transform; this was not the case (Table 2). Megalopae exposed to oil dispersed with
Corexit 9527 had the lowest transformation rate, especially among those organisms
that survived the 48-h exposure period.
Discussion
Results from the trials involving dispersants (Corexit 9500 and Corexit 9527)
and dispersed oil revealed higher toxicity of the dispersed oil relative to the
dispersant alone. Dispersants allow easier uptake and ingestion of the crude oil
via breakdown and dissolution of the oil into the water column (George-Ares
and Clark 2000, Ramachandran et al. 2004). Dispersed oil also had a larger impact
on life-stage transitioning than did the crude oil or dispersant alone. Both
Corexit 9500 and 9527 were used during the 2010 Deepwater Horizon incident;
Corexit 9500 was applied at both the wellhead and the surface, and Corexit 9527
was applied the surface only (Kujawinski et al. 2011). Corexit 9500 did not increase
the toxicity of the oil as much as did Corexit 9527 (48-h LC50 51.83 mg/L
vs 29.83 mg/L) and it did not impact life-stage transitioning as severely (77.5%
vs 1.7% transformed). The 9500 formula was also less toxic alone than the 9527
formula (48-h LC50 = 59.09 mg/L vs 37.46 mg/L). Our results suggest that toxicity
effects on Blue Crab larval populations might be reduced if Corexit 9500
rather than over Corexit 9527 is used as a dispersant for future oil releases in marine
environments. However, other studies have found increased toxicity of crude
oil treated with Corexit 9500 when compared to Corexit 9527 in Hydra viridissima
Pallas (Green Hydra) and similar toxicity of 9500 and 9527 in Haliotis
rufescens Swainson (Red Abalone) and Mysid holmesimysis Holmes (Kelp Forest
Mysid) (Mitchell and Holdway 2000, Singer et al. 1996). Acute aquatic toxicity
of selected dispersants must be considered in conjunction with targeted species,
environmental conditions, and application concentration and treatment location
because toxicity varies widely according to species, larval stage, and treatment
methodology (George-Ares and Clark 2000)
Life-stage transitioning
Trials examining toxicity of dispersed oil can provide further insight by incorporating
life-stage transitioning responses in the larvae. High transformation ratios
(alive:deceased) indicate more-acute toxic effects of the solution on the organism.
For instance, in trials involving of Corexit 9527-dispersed oil, only 1.7% of larvae
metamorphosed during exposure (Table 2). More specifically, 20% of organisms
that did not survive the exposure transformed before dying and only 5.3% of organisms
that survived achieved metamorphosis. All larvae in control treatments
transformed during the 48-h period. The treatment effects on life-stage transitioning
and mortality occurrence before or after metamorphosis are outlined in Table 2.
As previously mentioned, these were not dose-response effects. Rather, the effects
suggest that exposure to any toxicant (however slight the dosage) influenced transformation
of the megalopae in experimental trials.
Southeastern Naturalist
G89
R. Fern, K. Withers, P. Zimba, T. Wood, and L. Schoech
2015 Vol. 14, No. 4
The megalopae that survived and did not metamorphose during the initial
48-h exposure period eventually transformed during the 48-h post-exposure period.
While these organisms can delay transformation in light of unfavorable
environmental conditions, they cannot delay it indefinitely (Forward et al. 1996).
Metamorphosis delay is physiologically costly and may manifest in certain sublethal
effects in later life stages, although further studies are needed to determine
these long-term effects in Blue Crabs (Gebauer et al. 1999). Also, fewer organisms
that died had metamorphosed during the 48-h exposure period than did the organisms
that survived. This result implies that Blue Crabs may be most sensitive to the
toxin during the actual metamorphosis. Additional investigations into the physiological
effects of these dispersants and dispersed oil are needed.
Although it is used often in smaller-scale terrestrial spills, MicroBlaze had not
yet been tested in a laboratory setting so neither its performance as a dispersant,
nor its relative toxicity to aquatic organisms was established prior to this study. Our
results suggest that while it does not appear to be a viable option for immediate
crude-oil dispersal, hydrocarbon-degradation effects occurring after 48 h of exposure
may make this product a potential bioremediation additive for future oil-spill
protocols; investigations into its utility are ongoing. Despite a slight depression of
metamorphosis, this microbial surfactant also has the advantage of being essentially
non-toxic.
Adaptable methods
We developed our method of assessing acute toxicity of a contaminant from a
standard copepod 48–96-h LC50 assay. The success of the control-lifespan trials in
the vials and the consistent mortality gradients of the reference toxicant and experimental
doses indicate that this method is well suited for determining acute toxicity
in megalopae. Our results also suggest that this method may be further adapted and
used for toxicity assays of other larval or planktonic organisms. The SDS reference
toxicant 48-h LC50 value found in this study (5.6 mg/L) differs from the published
value (9.8 mg/L) for Blue Crab megalopae. As previously mentioned, the Whiting
et al. (1996) study used larvae in an earlier life stage that may have different physiological
requirements. The megalopae used in this study underwent an important
and physiologically demanding transition in which the first carapace is developed
and the telson undergoes fusion. The increase in physiological demands during
metamorphosis may have caused the increase in sensitivity to the toxin. Additionally,
the Whiting et al. (1996) study grouped the larvae together and exposed the
organisms to the toxicant in open, 250-mL glass containers. In our experiments, we
isolated each larva and exposed each organism in a separate, sealed 25-mL vial.
The lack of dilution, evaporation, and interaction of other larvae with the toxicant
may also have contributed to the higher toxicity. The lower value of 5.9 mg/L was
consistent across all reference toxicant trials (S = 0.62).
Study relevance and limitations
The purposes of this study were to determine acute toxicity of dispersants commonly
used in oil-spill response protocols, evaluate the potential of megalopae as
Southeastern Naturalist
R. Fern, K. Withers, P. Zimba, T. Wood, and L. Schoech
2015 Vol. 14, No. 4
G90
a model laboratory organism in toxicological studies, and establish any acute sublethal
effects of these toxicants on Blue Crab megalopae, especially on life-stage
metamorphosis. We did not address the physiological mechanism by which toxicity
occurs. Future studies that describe those mechanisms could provide even further
insight into the toxicological effects of oil and dispersants in the natural environment.
Conclusions drawn from our data should only be applied to laboratory settings
in which environmental variables are kept constant; toxicity of these chemicals can
be affected by temperature and salinity (NRC 1989). After establishing the basic
48-h LC50 values for the dispersants and dispersed oil, experiments like this study
should be performed under various temperature and salinity conditions to further
understand how these dispersants interact with oil and aquatic organisms in natural
settings. Additionally, in a natural landscape, water turbulence, UV-ray exposure
and currents would all influence the exposure of the toxicants to organisms in the
water column (Garrett et al 1998, Pace et al 1995). Concentrations of and exposure
periods to dispersants, crude oil, and dispersed oil found in natural settings should
be determined via water sampling or a larger scale mesocosm study capable of
mimicking open-ocean forces on water-column chemistry. This study establishes
baseline 48-h LC50 values for each of these dispersants alone and in combinations
with crude oil that would facilitate future investigation into real-world exposure of
these animals in various oil-spill scenarios.
Acknowledgments
We acknowledge the Center for Coastal Studies at Texas A&M University-Corpus
Christi for the use of their laboratory space and equipment. Credit should be given to the
National Spill Control School at Texas A&M University-Corpus Christi for their supply of
crude oil and dispersants, and to Lindsey George for her assistance in field collection and
lab work. This research project received no specific grant from any funding agency in the
public, commercial, or not-for-profit sectors. However, MicroBlaze-Verde Environmental,
Inc., Houston, TX provided support exclusively for transportation expenses to and from the
collection site for the primary investigator during a portion of the project.
Literature Cited
Ahsanullah, M., and G.H. Arnott. 1978. Acute toxicity of copper, cadmium, and zinc to larvae
of the crab Paragrapsus quadridentatus (H. Milne Edwards), and implications for
water-quality criteria. Australian Journal of Marine and Freshwater Research 29:1–8.
Atlas, R.M. 1981. Microbial degradation of petroleum hydrocarbons: An environmental
perspective. Microbiology Review 45:180–209.
Bik, H.M., K.M. Halanych, J. Sharma, and W.K Thomas. 2012. Dramatic shifts in benthic
microbial eukaryote communities following the Deepwater Horizon oil spill. PLoS ONE
7, e38550. doi:10.13771/journal.pone.0038550
Brakstad, O.G. 2008. Natural and simulated biodegradation of petroleum in cold marine
environments. Pp. 447–473, In R. Margesin, F. Schinner, J.C. Marx, and C. Gerday
(Eds.). Psychrophiles: From Biodiversity to Biotechnology. Springer Verlag, Berlin,
Germany. 462 pp.
Churchill, E.P., Jr. 1919. Life history of the Blue Crab. Bulletin of the Bureau of Fisheries
36:95–128.
Southeastern Naturalist
G91
R. Fern, K. Withers, P. Zimba, T. Wood, and L. Schoech
2015 Vol. 14, No. 4
Conner, P.M. 1972. Acute toxicity of heavy metals to some marine larvae. Marine Pollution
Bulletin 3:190–192.
Couillard, C.M., K. Lee, B. Legare, and T.L. King. 2005. Effect of dispersant on the composition
of the water-accommodated fraction of crude oil and its toxicity to larval marine
fish. Environmental Toxicology and Chemistry 24:1496–1504.
Dubansky, B., A. Whitehead, J. Miller, C.D. Rice, and F. Galvez. 2013. Multi-tissue molecular,
genomic, and developmental effects of the Deepwater Horizon oil spill on resident
Gulf Killifish (Fundulus grandis). Environmental Science and Technology doi:10.1021/
es400458p.
Duke, N.C., K.A. Burns, R.P.J. Swannell, O. Dalhaus, and R.J. Rupp. 2000. Dispersant use
and a bioremediation strategy as alternate means of reducing impacts of large oil spills
on mangroves: The Gladstone Field trials. Marine Pollution Bulletin 41:403–412.
Forward, R.B., M.C. DeVries, D. Rittschof, D.A.Z. Frankel, J.P. Bischoff, C.M Fisher, and
J.M. Welch. 1996. Effects of environmental cues on metamorphosis of the Blue Crab,
Callinectes sapidus. Marine Ecology Progress Series 131:165–177.
Garrett, R.M., I. Pickering, C. Haith, and R. Prince. 1998. Photooxidation of crude oils.
Environmental Science and Technology 32:3719–3723.
Gebauer, P., K. Paschke, and K. Anger. 1999. Costs of delayed metamorphosis: Reduced
growth and survival in early juveniles of an estuarine grapsid crab, Chasmagnathus
granulata. Journal of Experimental Marine Biology and Ecology 238:271–281.
George-Ares, A.G., and J.R. Clark. 2000. Aquatic toxicity of two Corexit dispersants. Chemosphere
40:897–906.
Getter, C.D., T.G. Ballou, and C.B. Koons. 1985. Effects of dispersed oil on mangroves:
Synthesis of a seven-year study. Marine Pollution Bulletin 16:318–324.
Gorbi, G., M. Invidia,F. Savorelli, O. Faraponova, E. Giacco, M. Cigar, I. Buttino, T. Leoni,
E. Prato, I., Lacchetti, and S. Sei. 1909. Standardized methods for acute and semichronic
toxicity tests with the copepod Acartia tonsa. Environmental Toxicology and Chemistry
31:2023–2028. doi:10.1002. Accessed 6 July 2013.
Jacobi, C.C., and K. Anger. 1985. Growth and respiration during the larval development of
Hyas coarctatus (Decapoda: Majidae). Marine Biology 87:173–180.
Kujawinski, E.B., M.C. Kido Soule, D.L. Valentine, A.K. Boysen, K. Longnecker, and M.C.
Redmond. 2011. Fate of dispersants associated with the Deepwater Horizon oil spill.
Environmental Science and Technology 45:1298–1306.
Lindstrom, J.E., and J.F. Braddock. 2002. Biodegradation of petroleum hydrocarbons at low
temperature in the presence of the dispersant Corexit 9500. Marine Pollution Bulletin
44:739–747.
Mangum, C.P., B.R. McMahon, P.L. Defur, and M.G. Wheatly. 1985. Gas exchange, acidbase
balance, and the oxygen supply of the tissues during a molt of the Blue Crab Callinectes
sapidus. Journal of Crustacean Biology 5:188–206.
Mitchell, F.M., and D.A. Holdway. 2000. The acute and chronic toxicity of the dispersants
Corexit 9527 and 9500, water accommodated fraction (WAF) of crude oil, and dispersant-
enhanced WAF (DEWAF) to Hydra viridissima (Green Hydra). Water Resources
34:343–348.
National Marine Fisheries Service (NMFS). 2012. Fisheries of the United States 2011. Current
Fishery Statistics Number 2011. Silver Spring, MD. Available online at http://www.
st.nmfs.noaa.gov/st1/fus/fus11/FUS_2011.pdf. Accessed 3 July 2013.
National Research Council (NRC). 1989. Using Oil-Spill Dispersants on the Sea. National
Academy Press, Washington, DC. 352 pp.
Southeastern Naturalist
R. Fern, K. Withers, P. Zimba, T. Wood, and L. Schoech
2015 Vol. 14, No. 4
G92
NRC. 2005. Oil-spill Dispersants: Efficacy and Effects. National Academy Press, Washington,
DC. 400 pp.
Pace, C.B., J.R. Clark, and G.E. Bragin. 1995. Comparing crude-oil toxicity under standard
and environmentally realistic exposures. Pp. 1002–1004, In Proceedings of the 1995
International Oil-Spill Conference. American Petroleum Institute, Washington, DC.
Perkins, E.J., E. Gribbon, and J. Logan. 1973. Oil-dispersant toxicity. Marine Pollution
Bulletin 4:90–93.
Ramachandran, S.D., P. Hodson, C. Khan, and K. Lee. 2004. Oil dispersant increases
PAH uptake by fish exposed to crude oil. Ecotoxicology and Environmental Safety
59:300–308.
Shanks, A.L. 1985. Behavioral basis of internal wave-induced shoreward transport of
megalopae of the crab Pachygrapsus crassipes. Marine Ecological Progress Series
24:289–295.
Singer, M.M., S. George, S. Jacobson, I. Lee, L.L. Weetman, R.S. Tjeerdema, and M.L.
Sowby. 1996. Comparison of acute aquatic effects of the oil dispersant Corexit 9500
with those of other Corexit-series dispersants. Ecotoxicology and Environmental Safety
45:183–189.
Sulkin, S.D., and W. Van Heukelem. 1982. Larval recruitment in the crab Callinectes sapidus
Rathbun: An amendment to the concept of larval retention in estuaries. Pp. 459–476, In
V.S. Kennedy (Ed.). Estuarine Comparisons. Academic Press, New York, NY. 1032 pp.
UnHyuk, Y., and H. GiMyeong. 2009. Biochemical changes in Rockfish, Sebastes
schlegeli, exposed to dispersed crude oil. Comparative Biochemistry and Physiology C
150:218–223.
Wells, P.G. 1984. The toxicity of oil spill dispersants to marine organisms: A current perspective.
Pp 177–202, In T.E. Allen (Ed.). Oil Spill Chemical Dispersants: Research,
Experience, and Recommendations. American Society for Testing and Materials, Philadelphia,
PA. 840 pp.
Whiting, V.K., G.M. Cripe, and J.E. Leop. 1996. Effects of the anionic surfactant, sodium
dodecyl sulfate, on newly-hatched Blue Crabs, Callinectes sapidus, and other routinely
tested estuarine crustaceans. Archives of Environmenatl Contamination and Toxicology
31:293–295.
Zuijdgeest, A., and M. Huettel. 2012. Dispersants as used in response to the MC252-spill
lead to higher mobility of polycyclic aromatic hydrocarbons in oil-contaminated Gulf of
Mexico sand. PLoS ONE 7, e50549. doi:10.1371/journal.pone.0050549.