Movement and Resource Selection of Baird’s Pocket Gopher
within a Longleaf Pine Ecosystem
Robert O. Wagner, Matthew B. Connior, Christopher A. Melder, Brett S. Cooper, Dwayne Hightower, and Sarah Pearce
Southeastern Naturalist, Volume 16, Issue 3 (2017): 397–410
Full-text pdf (Accessible only to subscribers.To subscribe click here.)
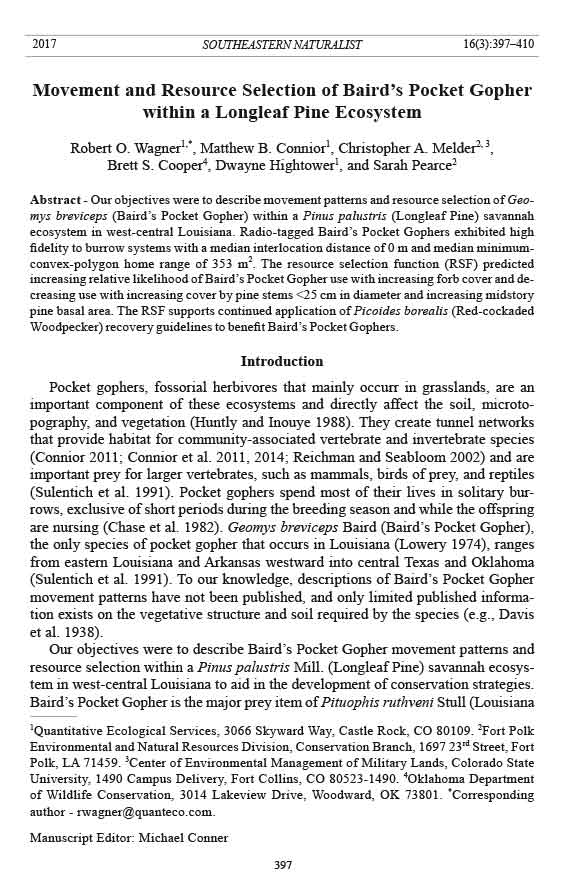
Southeastern Naturalist
397
R.O. Wagner, M.B. Connior, C.A. Melder, B.S. Cooper, D. Hightower, and S. Pearce
22001177 SOUTHEASTERN NATURALIST 1V6o(3l.) :1369,7 N–4o1. 03
Movement and Resource Selection of Baird’s Pocket Gopher
within a Longleaf Pine Ecosystem
Robert O. Wagner1,*, Matthew B. Connior1, Christopher A. Melder2, 3,
Brett S. Cooper4, Dwayne Hightower1, and Sarah Pearce2
Abstract - Our objectives were to describe movement patterns and resource selection of Geomys
breviceps (Baird’s Pocket Gopher) within a Pinus palustris (Longleaf Pine) savannah
ecosystem in west-central Louisiana. Radio-tagged Baird’s Pocket Gophers exhibited high
fidelity to burrow systems with a median interlocation distance of 0 m and median minimumconvex-
polygon home range of 353 m2. The resource selection function (RSF) predicted
increasing relative likelihood of Baird’s Pocket Gopher use with increasing forb cover and decreasing
use with increasing cover by pine stems less than 25 cm in diameter and increasing midstory
pine basal area. The RSF supports continued application of Picoides borealis (Red-cockaded
Woodpecker) recovery guidelines to benefit Baird’s Pocket Gophers.
Introduction
Pocket gophers, fossorial herbivores that mainly occurr in grasslands, are an
important component of these ecosystems and directly affect the soil, microtopography,
and vegetation (Huntly and Inouye 1988). They create tunnel networks
that provide habitat for community-associated vertebrate and invertebrate species
(Connior 2011; Connior et al. 2011, 2014; Reichman and Seabloom 2002) and are
important prey for larger vertebrates, such as mammals, birds of prey, and reptiles
(Sulentich et al. 1991). Pocket gophers spend most of their lives in solitary burrows,
exclusive of short periods during the breeding season and while the offspring
are nursing (Chase et al. 1982). Geomys breviceps Baird (Baird’s Pocket Gopher),
the only species of pocket gopher that occurs in Louisiana (Lowery 1974), ranges
from eastern Louisiana and Arkansas westward into central Texas and Oklahoma
(Sulentich et al. 1991). To our knowledge, descriptions of Baird’s Pocket Gopher
movement patterns have not been published, and only limited published information
exists on the vegetative structure and soil required by the species (e.g., Davis
et al. 1938).
Our objectives were to describe Baird’s Pocket Gopher movement patterns and
resource selection within a Pinus palustris Mill. (Longleaf Pine) savannah ecosystem
in west-central Louisiana to aid in the development of conservation strategies.
Baird’s Pocket Gopher is the major prey item of Pituophis ruthveni Stull (Louisiana
1Quantitative Ecological Services, 3066 Skyward Way, Castle Rock, CO 80109. 2Fort Polk
Environmental and Natural Resources Division, Conservation Branch, 1697 23rd Street, Fort
Polk, LA 71459. 3Center of Environmental Management of Military Lands, Colorado State
University, 1490 Campus Delivery, Fort Collins, CO 80523-1490. 4Oklahoma Department
of Wildlife Conservation, 3014 Lakeview Drive, Woodward, OK 73801. *Corresponding
author - rwagner@quanteco.com.
Manuscript Editor: Michael Conner
Southeastern Naturalist
R.O. Wagner, M.B. Connior, C.A. Melder, B.S. Cooper, D. Hightower, and S. Pearce
2017 Vol. 16, No. 3
398
Pinesnake) (Rudolph et al. 2002, 2012)—a species proposed for listing under the
Endangered Species Act of 1973 (16 U.S.C. § 1531 et seq.)2; thus, maintaining adequate
populations of Baird’s Pocket Gophers is critical to maintaining Louisiana
Pinesnake populations.
Field-site Description
Our study area was limited to the Fort Polk Military Installation and the northern
half of the Vernon Unit of the Kisatchie National Forest in west-central Louisiana.
We used the model of Louisiana Pinesnake soil suitability developed by Wagner et
al. (2014) and historic localities of Baird’s Pocket Gophers from Fort Polk’s database
of mound-complex locations to define our study area within those boundaries.
The model identified sandy, well-drained soils as preferred habitat for Louisiana
Pinesnakes and most (339 of 342, or 99%) Baird’s Pocket Gopher mound-complex
locations on Fort Polk and the Vernon Unit occurred on soils suitable for the Louisiana
Pinesnake. Forests within the study area were predominantly uneven-aged
managed Longleaf Pine savanna with a sparse mid-story. Herbaceous cover was
prevalent and maintained by prescribed fires every 2–5 years.
Methods
Movements
To study Baird’s Pocket Gopher movement patterns, we trapped and radiotagged
pocket gophers at mound complexes during December 2013. We consulted
Fort Polk’s database of mound-complex locations to locate those that were active.
All animal handling and tagging procedures were consistent with the live-animal
handling guidelines of the American Society of Mammalogists (Gannon et al.
2007). We used live boxtraps to capture pocket gophers (Connior and Risch 2009)
at selected mound complexes. We placed traps inside the burrows; covered the tops
with dirt, plywood, or both; and checked them every ½–1 hour. We anesthetized
Baird’s Pocket Gophers with ether and recorded age, sex, length, weight, and location.
We implanted subcutaneous radio transmitters (either SOPI-2190 [4–6 g, 237-
day battery life]; SOPI-2070 [2–3 g, 139-day battery life]; or SOPI-2038 [1.8–2.2
g, 86-day battery life]; Wildlife Materials Inc., Murphysboro, IL) into individuals
following the procedures described in Connior and Risch (2010). For each Pocket
gopher, we inserted the largest transmitter possible, but transmitters were never >5
% of the animal’s body mass (Connior and Risch 2010). To assist with animal identification
in the event of transmitter loss or failure, we inserted passive integrated
transponder (PIT) tags into all radio-tagged individuals. We returned all animals to
their burrows within an hour of transmitter placement.
We attempted to relocate radio tagged individuals twice per week between 16
January 2013 and 8 June 2014, typically between 10:20 and 12:42 (though as early
as 5:10 and as late as 21:43) using a TRX-1000s receiver (Wildlife Materials Inc.,
Murphysboro, IL) and a model F-172 handheld H antenna (AF Antronics, Inc.,
Urbana, IL). We relocated animals using homing procedures described by Samuel
Southeastern Naturalist
399
R.O. Wagner, M.B. Connior, C.A. Melder, B.S. Cooper, D. Hightower, and S. Pearce
2017 Vol. 16, No. 3
and Fuller (1994) and marked each animal’s subterranean location within its mound
complex by inserting a pin flag into the soil. We estimated the location using GPS
(Garmin GPS map 76CSX and Oregon 550, Garmin International, Inc., Olathe,
KS) by averaging a minimum of 60 WAAS-corrected locations. If no discernable
movement had occurred since the prior location was recorded, we left the pin flag
in place and used the initial GPS location. We assessed GPS location precision by
collecting thirty 60-location averages at a fixed location with the GPS units used
in the study. Accuracy assessment locations were spaced a minimum of 2 h apart
and were collected on consecutive days under conditions similar to those found at
Baird’s Pocket Gopher locations. We defined precision as the distance root mean
square error (dRMSE [expected to capture ~63% of the locations] and 2dRMSE
expected to capture 95% of the locations]) averaged across GPS units (n = 3).
To describe shorter-duration Baird’s Pocket Gopher movements, we estimated
interlocation distances, or the distance between consecutive relocations, which
we summarized for each animal as the fraction of relocations without detectable
movement and median distance moved between relocations. To describe movement
patterns over the study’s duration, we estimated individual home ranges as
minimum convex polygons (MCP) using Geospatial Modeling Environment (Beyer
2015, R Core Team 2015).
Animals might move greater distances over longer periods of time; thus, we
compared the mean number of days between relocations by sex and used Spearman’s
correlation estimates to evaluate the influence of interlocation interval on
distance moved. Similarly, because MCPs have been observed to increase with
animal body mass for other gopher species (e.g., female G. bursarius ozarkensis
Elrod, Zimmerman, Sudman and Heidt [Ozark Pocket Gopher]; Connior and Risch
2010) and could increase with increasing numbers of animal locations, we used
Spearman’s correlation estimates to evaluate the relationships between MCPs and
Baird’s Pocket Gopher mass and number of locations. We tested for differences
between sexes in movement metrics using Wilcoxon rank-sum tests because those
metrics were not normally distributed. All statistical comparisons except resource
selection modeling were performed using S-Plus statistical software (TIBCO
Software, Inc., Palo Alto, CA), and we employed a Type I error rate of 5% for all
statistical tests.
Resource selection
To construct resource selection functions (RSF; Johnson et al. 2006, Manly et
al. 2002) for Baird’s Pocket Gophers, we selected separate samples from sites with
(used) and without (unused) active pocket gopher mound complexes. We sampled
unused resources throughout the study area and contrasted those data with a sample
of used resources, equivalent to the Manly et al. (2002) sampling protocol C (SPC),
design I.
We selected used sites randomly (n = 41) from 342 sites known to be occupied
via surveys conducted between October 2011 and September 2013, and recorded in
Fort Polk’s pocket gopher mound-complex database. We defined mound complexes
Southeastern Naturalist
R.O. Wagner, M.B. Connior, C.A. Melder, B.S. Cooper, D. Hightower, and S. Pearce
2017 Vol. 16, No. 3
400
as continuous distributions of mounds uninterrupted by improved surface roads,
waterways, or 125 m of unoccupied habitat (the maximum tunnel length reported
by Wilkins and Roberts [2007] for Baird’s Pocket Gopher). Most mound complexes
were a collection of 2 or more separate tunnel networks, and we assumed that each
tunnel network was occupied by a single gopher due to their solitary nature (Chase
et al. 1982). To sample unused resources, we selected random locations within the
study area and conducted site visits to determine Baird’s Pocket Gopher occupancy.
The unused sample was comprised of data from the first 25 unoccupied random
sites encountered that remained unused throughout the study period as confirmed
by periodic visits.
We compiled a list of resources potentially important to Baird’s Pocket Gophers,
similar to those presented by Himes et al. (2006), that included edaphic factors,
vegetation-cover measures, and woody-debris metrics. Edaphic factors were soil
texture, slope, and aspect. We measured vegetation cover between September 2013
and December 2014 in the following strata: herbaceous (all vegetation less than 2 m in
height), mid-story (trees >2 m tall and to tree canopy), and over-story (tree canopy).
At used sites, we visually estimated the center of the mound complex and recorded
the location using GPS to establish the plot center, and we used the randomly chosen
GPS coordinates for unused sites.
We estimated edaphic factors using available digital data and field measurements.
We recorded soil composition as the percent of sand, clay, and loam as
determined from a 0.75-m-deep sample collected at plot center. We used ArcMap
(ESRI, Redlands, CA) to estimate slope (%) from the National Elevation Dataset
(USGS 2016) and measured aspect in degrees using a handheld compass.
Within the herbaceous stratum, we visually estimated percent woody stems,
grasses, forbs, bare ground, and litter at a single 1-m-radius plot around the plot
center. We collected 4 measures of mid-story cover: (1) basal area of mid-story
pine trees, (2) basal area of hardwood mid-story trees, (3) visibility measured as
a percent of a 1.9-m-tall (six 1-ft increments and 3-inch tip) and 3.8-cm (1½ in)
diameter Robel pole placed 21.3 m (70 ft) in a random direction from the plot
center, and (4) the number of stems less than 25 cm (10 in) DBH counted within a 5-m
radius around the plot center. We used the basal area of overstory trees ≥25 cm to
estimate over-story cover and measured percent canopy closure with a concave
spherical densiometer (Lemmon 1957; Forest Densiometers, Rapid City, SD) at
the 4 cardinal directions from plot center and averaged the values. Mid-story and
over-story basal areas were measured at plot center using a 1-factor metric prism
as m2/hectare. To characterize woody debris, we determined the number of pine
and hardwood logs >10 cm and the number of standing snags and stumps within
an 11.2-m radius of plot center.
We could not locate published studies of Baird’s Pocket Gopher’s resource
selection to guide our model-development process. Without a priori knowledge of
variable importance in gopher habitat selection, we used R (R Development Core
Team 2016) to perform the exploratory data-analysis procedure of considering all
possible unique models constructed from all complete (no missing data) candidate
Southeastern Naturalist
401
R.O. Wagner, M.B. Connior, C.A. Melder, B.S. Cooper, D. Hightower, and S. Pearce
2017 Vol. 16, No. 3
variables. We used automated model selection (R Package glmulti, Calcagno 2015)
to identify the best logistic regression model for habitat-quality prediction and
determination of variable importance. We defined the best model as the one that
minimized Akaike’s information criterion adjusted for small sample size (AICc;
Burnham and Anderson 1998), and included only important variables.
The number of models considered was large relative to the available data, which
increased the likelihood of including spurious effects; small sample size increased
the likelihood of missing important effects (Calcagno and de Mazancourt 2010).
To reduce the likelihood of including unimportant variables in the best model and
ensure inclusion of all important variables, we examined across-model variable importance
(or relative evidence weight). Variable importance is computed as the sum
of the relative evidence weights of all models in which the variable appears. We
chose an across-model variable-importance threshold of 80% to classify all candidate
variables. We considered to be unimportant all variables with an importance
score less than the threshold and eliminated them from the model with the lowest
AICc (Calcagno and de Mazancourt, 2010).
Following selection of the best model, we performed a series of diagnostic
tests. We compared the best model to the null model using a likelihood ratio test
(LRT) as a secondary test of model fit. We evaluated the best model for evidence of
non-linearity of the logit by examining the best-model residuals and the presence
of overly influential observations using DFBETAS and DFITS (Harrell 2001). We
used LRT to assess additivity of model variables by testing the best model against
a comparable model with all 2-way interactions. Model predictive ability was assessed
using concordance, c, which is identical to the receiver operating curve
(ROC), with values greater than 0.8 considered as having utility in predicting the
response of individual subjects (Harrell 2001). Lastly, we assessed relative importance
of variables in the best model by determining their proportionate contribution
to the overall model chi-square (R package rms; Harrell 2017).
We selected separate samples of used and unused resources with unknown
sampling probabilities; thus, reliable estimation of β0, the model intercept, was
impossible. Consequently, the RSF was an estimated value rather than a resource
selection probability function (Manly et al. 2002). We used the coefficients β1 - βn
of the best model to estimate an exponential function of the relative probability of
selection (wi, where wi = exp[β1x1 + … + βnxn]) by Baird’s Pocket Gophers (Johnson
et al. 2006, Manly et al. 2002).
We compared the distribution of estimated wi for used and unused sites to interpret
our results. We set the threshold above which resources would be estimated to
be used halfway between the 75th percentile wi for unused sites and the 25th percentile
wi for used sites; the percentage of observed data that was correctly classified
using the threshold was determined (e.g., sites correctly classified as used = [n of
used sites with wi ≥ threshold] / [n used sites] * 100). To provide a quantitative
description of habitat conditions at used and unused sites, we calculated means and
standard errors for candidate variables.
Southeastern Naturalist
R.O. Wagner, M.B. Connior, C.A. Melder, B.S. Cooper, D. Hightower, and S. Pearce
2017 Vol. 16, No. 3
402
Table 1. Radio-tagged Baird’s Pocket Gopher movement metrics by sex (female = 5, male = 8) based
on twice weekly relocations (16 January 2013–8 June 2014) within the Fort Polk-Vernon Unit study
area. Interlocations = distance between consecutive relocations.
Percent interlocations Median interlocation
Metric without movement distance (m) MCP (m2)
Sex F M All F M All F M All
Median 56 49 51 0.0 1.1 0.0 139.0 388.0 353.0
Mean 47 45 4 1.8 3.4 2.7 270.6 620.8 486.1
SE 12 6 6 1.5 1.8 1.2 162.2 203.5 143.7
Results
Movement
Mound complexes were typically occupied by multiple individuals. We captured
more than 1 Baird’s Pocket Gopher (2–5) at 4 of the 6 mound complexes included
in the movement study and radiotagged a total of 14 Baird’s Pocket Gophers—6 females
and 8 males. One female was documented at only 2 locations and was dropped
from movement analysis. For the remaining 13 Baird’s Pocket Gophers, 395 interlocation
distances (female = 133, male = 262) were calculated from 408 locations (GPS
dRMSE = 7.8 m). Interlocation distances within animals, animal specific median
interlocation distances, and MCPs were right-skewed. Female (mean = 79.0 g, SE =
3.5 g) and male (mean = 104.8 g, SE = 9.9 g) mass did not differ (Z = -1.83, P = 0.07).
Mean number of days between relocations was the same for females (mean = 4.2,
female SE = 0.17) and males (mean = 4.3, female SE = 0.19), and did not influence
interlocation distance (Z = -0.02, P = 0.98). Number of locations was not correlated
with MCP size (females: Z = 0.11, P = 0.92; males: z = 1.61, P = 0.11).
Across all female locations, interlocation distances ranged from 0 m to 52.6 m
(median = 0 m), and 0 m to 71.2 m across male locations (median = 2.2 m). The
fraction of locations without movement (W = 37, P = 0.83), median interlocation
distance (Z approximation = -0.45, P = 0.65), and MCP (W = 22, P = 0.07) did not
differ between males and females (Table 1).
Resource selection
Soil samples were not collected at all plots (used: n = 40; unused, n = 14); thus,
soil composition was not included in the set of candidate variables for RSF development.
Although the study area was constrained to sandy, well-drained soils, the
available data suggested that used sites had a greater percentage of sand (used:
mean = 65.8, SE = 0.3; unused: mean = 55.7, SE = 0.6; t = 3.17, P < 0.01) and lower
percent of loam (used: mean = 24.5, SE = 0.3; unused: mean = 33.6, SE = 0.9; t =
-2.4.17, P = 0.02) than unused sites. Clay content did not differ (t = -0.56, P = 0.58).
All other candidate variables (n = 20; Table 2) were complete (no missing data) and
were included in the set of candidate variables for RSF development.
Automated model selection identified the logistic regression model containing
percent forb groundcover, visibility, number of stems less than 25 cm, and midstory
pine basal area as having the minimum AICc of all possible models. All variables
Southeastern Naturalist
403
R.O. Wagner, M.B. Connior, C.A. Melder, B.S. Cooper, D. Hightower, and S. Pearce
2017 Vol. 16, No. 3
Table 2. Comparison of habitat measures between used and unused Baird’s Pocket Gopher study sites,
collected between September 2013 and December 2014, within the Fort Polk-Vernon Unit study area.
Unused (n = 25) Used (n = 41)
Variable Mean SE Mean SE
Woody groundcover (%) 11.05 2.35 5.51 0.96
Grass groundcover (%) 24.38 3.68 31.70 2.73
Forb groundcover (%) 14.71 2.16 23.84 1.89
Bare groundcover (%) 19.86 3.91 20.49 2.24
Litter groundcover (%) 62.40 5.53 53.17 3.63
Slope (%) 2.67 0.27 3.01 0.35
Aspect (°) 145.99 21.65 173.70 16.37
Total logs (count) 14.00 1.70 11.90 2.23
Pine logs (count) 9.72 1.55 10.78 2.26
Hardwood logs (count) 4.28 1.73 1.12 0.41
Total basal area 20.94 2.38 13.34 1.72
Canopy pine basal area (m2/ha) 14.88 2.17 11.25 1.47
Canopy hardwood basal area (m2/ha) 3.54 0.93 1.32 0.68
Midstory pine basal area (m2/ha) 2.34 0.80 0.59 0.26
Midstory hardwood basal area (m2/ha) 0.18 0.11 0.18 0.10
Canopy closure (%) 69.92 5.16 55.31 3.85
Visibility (% rod visible) 65.79 3.56 75.23 2.32
Stems less than 5 cm DBH (count) 35.24 6.26 11.78 2.68
Snags (count) 0.40 0.17 0.37 0.11
Stumps (count) 1.40 0.47 1.88 0.38
Table 3. Coefficients for Baird’s Pocket Gopher resource selection function based on the best model.
The best model, defined as the one that minimizes AICc and includes only important variables, was
selected from all possible combinations of 20 variables measured between September 2013 and December
2014, within the Fort Polk-Vernon Unit study area. A variable was considered important if
the sum of relative evidence weights across all models in which the variable appeared exceeded 80%.
Variable Value SE Wald Z P
Forb groundcover (%) 0.0878 0.0315 2.78 0.005
Stems less than 25 cm DBH -0.0384 0.0163 -2.36 0.018
Midstory pine basal area -0.2617 0.1572 -1.66 0.096
included in the model with the lowest AICc had an across-model variable importance
(Fig. 1) greater than the 80% threshold, except visibility. When we removed
visibility, the best model included percent forb groundcover, number of stems
less than 25 cm, and midstory pine basal area. Evaluation of the best model provided no
evidence of non-linearity of the logit, overly influential observations, or lack of additivity
(P = 0.92). The best model fit the data well (model LRT χ2 = 26.3, df = 3, P less than
0.0001), and had predictive ability (c = 0.836). Variable rank in order of importance
within the best model was: percent forb groundcover, number of stems less than 25 cm, and
midstory pine basal area (Fig. 2).
We estimated the RSF wi using the best model coefficients (Table 3). Based
on the RSF, the relative probability of selection increased with increasing forb
cover and decreased with increasing stems and midstory pine basal area (Table 4,
Southeastern Naturalist
R.O. Wagner, M.B. Connior, C.A. Melder, B.S. Cooper, D. Hightower, and S. Pearce
2017 Vol. 16, No. 3
404
Table 4. Estimated Baird’s Pocket Gopher resource selection function values (wi) under a range of
observed conditions at used and unused sites. Conditions are described as poor, median, and good for
used and unused sites based on the 25th, median, and 75th percentiles of observed conditions at used
and unused sites within the Fort Polk-Vernon Unit study area.
Variable value (percentile)
Resource condition Forb groundcover Stems less than 25 cm DBH Midstory pine basal area wi
Unused-poor 4 (25) 46 (75) 2.30 (75) 0.1
Unused-median 15 (50) 28 (50) 1.15 (50) 0.9
Unused-good 21 (75) 15 (25) 0.00 (25) 3.5
Used-poor 18 (25) 17 (75) 0.00 (75) 2.5
Used-median 22 (50) 6 (50) 0.00 (50) 5.5
Used-good 33 (75) 0 (25) 0.00 (25) 18.1
Figure 1. Across-model variable importance, computed as the sum of the relative evidence
weights of all models in which the variable appears. Models were sorted in ascending AICc
order and only variables appearing in the set of models with an accumulated evidence
weight of 95% are shown. Variables with importance values less than the 80% vertical reference
line were considered unimportant.
Southeastern Naturalist
405
R.O. Wagner, M.B. Connior, C.A. Melder, B.S. Cooper, D. Hightower, and S. Pearce
2017 Vol. 16, No. 3
Figure 2. Importance of variables in the best model, assessed as their proportionate contribution
to overall model chi-square. Chi-square and associated P-values are included for each variable.
Fig. 3). The midpoint between the 75th percentile wi for unused sites and the 25th
percentile wi for used sites was 1.97, which we rounded to 2. We classified sites
with an estimated relative probability of selection less than 2 as unused and all others as
used. The selected threshold resulted in 76% of used and unused sample sites correctly
classified.
Discussion
Due to the high energy expenditure of burrowing (Vleck 1979), pocket gophers
tend to limit movement once a burrow has been constructed. When we relocated
radio-tagged Baird’s Pocket Gophers, it was common (46%) to find the animal in
the same location on consecutive relocation events, suggesting fidelity to a suspected
daytime bedding or nest site. When we detected movement of an individual, the
distance moved was on average 2.7 m. Small distances moved between relocations
resulted in small average (across both sexes) home ranges (486.1 m²) and high siteSoutheastern
Naturalist
R.O. Wagner, M.B. Connior, C.A. Melder, B.S. Cooper, D. Hightower, and S. Pearce
2017 Vol. 16, No. 3
406
Figure 3. Relationship of
estimated Baird’s Pocket
Gopher resource selection
function wi to each variable
in the best model holding
the remaining variables to
their sample median; number
of stems less than 25 cm = 11.5,
percent forb cover = 19.8,
and midstory pine basal
area (m2/ha) = 0. In each
graph,the horizontal reference
line (dotted line) is the
threshold above which sites
are expected to be used and
below which sites are expected
to be unused.
fidelity, as reported by King (2010) for Baird’s Pocket Gophers in Arkansas. King
(2010) reported a mean distance of 18 m between recaptures of individuals within
their home range over the course of up to 29 months, with a maximum of 46 m.
Average MCP home-range sizes for females and males were 270.6 m² and
620.8 m², respectively. This is the first report of home ranges calculated for Baird’s
Pocket Gophers; thus, we cannot compare our estimates to any previously published
Southeastern Naturalist
407
R.O. Wagner, M.B. Connior, C.A. Melder, B.S. Cooper, D. Hightower, and S. Pearce
2017 Vol. 16, No. 3
conspecific estimates in similar habitat. However, our estimates were smaller than
home-range estimates of 921 m² reported for G. pinetis Rafinesque (Southeastern
Pocket Gopher) in a Longleaf Pine ecosystem in Georgia (Warren 2014). Larger
home-ranges for males than for females have also been reported in Ozark Pocket
Gopher (Connior and Risch 2010) and G. attwateri Merriam (Attwater’s Pocket Gopher;
Wilks 1963); these results may be related to reproductive opportunities.
Warren (2014) reported that soil characteristics had greater influence on Southeastern
Pocket Gopher habitat use than did vegetation characteristics in Longleaf
Pine forests of southwestern Georgia. We were unable to evaluate soil characteristics
in our habitat assessment because we had no soil samples from many unused
sample sites. Despite constraining the study area to sandy well-drained soils, the
soil samples collected as part of this study suggested that sites used by Baird’s
Pocket Gophers had a greater percentage sand and lower percentage loam than
unused sites.
When we evaluated only vegetation characteristics, the resource selection function
suggested that the relative probability of Baird’s Pocket Gopher use within
upland pine habitats on Fort Polk and the Vernon Unit increased with increasing
forb cover, and the relative probability of use decreased with increasing numbers of
tree stems < 25 cm DBH and increasing midstory pine basal area. Identification
of forb cover as the most important variable predicting Baird’s Pocket Gopher
habitat selection was consistent with the species’ preferences for herbaceous roots,
tubers, and stems (Sulentich et al. 1991), and others have reported that Geomys
bursarius (Shaw) (Plains Pocket Gopher) prefer to forage on forbs, especially
during the growing seasons (Luce et al. 1980, Myers and Vaughan 1964). Greater
densities of tree stems less than 25 cm DBH and greater midstory pine basal area increase
the occurrence of large, thick woody roots, which increases the difficulty of tunnel
excavation and reduces the likelihood of Baird’s Pocket Gopher use.
The upland pine habitats within the study area were predominately uneven aged
Longleaf Pine forest that are prescribed burned every 2–5 y. We caution against applying
this study’s results to forests with conditions that differ substantially from
the study area. Habitat characteristics determined to be unimportant on Fort Polk,
such as percent grass cover and canopy closure, could influence Baird’s Pocket
Gopher selection elsewhere.
Our motivation was to identify Baird’s Pocket Gopher movement patterns and
habitat requirements to aid in the development of conservation strategies for this
species, and consequently, the Louisiana Pinesnake. Baird’s Pocket Gophers exhibited
high site-fidelity, and multiple individuals frequently occurred within a
complex. Together, those factors suggest that once a Louisiana Pinesnake locates
a mound complex, it has the potential for many prey items and a burrow system
for protection.
Our habitat analysis indicated that maintenance of upland forest consistent with
forest management goals for the Red-cockaded Woodpecker, as described in the species’
recovery plan (USFWS 2003), will maintain suitable habitat for Baird’s Pocket
Gophers. Over the past decade the study area was managed in accordance with the
Southeastern Naturalist
R.O. Wagner, M.B. Connior, C.A. Melder, B.S. Cooper, D. Hightower, and S. Pearce
2017 Vol. 16, No. 3
408
Red-cockaded Woodpecker recovery plan. Specifically, prescribed fire was applied
every 2–5 y to reduce litter, woody debris, and other fuels, as well as promote herbaceous
growth and retard the growth of hardwood trees. Timber harvests promoted
open, park-like conditions and retention of the oldest trees and a density of less than 2.3
m2/ha (10 ft2 / acre) of pine stems less than 25 cm (10 in) DBH and a sparse hardwood midstory.
The selected RSF supports continued application of those guidelines to benefit
Baird’s Pocket Gophers and the associated Louisiana Pinesnake.
Acknowledgments
This research would not have been possible without the support of Fort Polk’s Environmental
and Natural Resources Management Division (ENRMD), and, in particular, C. Stagg
and W. Farris. We thank ENRMD Conservation Branch field crew members S. Carnahan,
R. Geroso, C. Allen, and S. Huskins for their work collecting and assembling the data used
in this study. M. Mouton, Natural Resource Conservation Service soil scientist, provided
guidance for soil sampling. We thank the Louisiana Department of Wildlife and Fisheries,
Natural Heritage Program for use of a Gopher Tortoise camera; the USDA Forest Service,
Kisatchie National Forest, for access to their property; and J. Sperry, Engineer Research and
Development Center research scientist, provided initial statistical support. We also thank 4
anonymous reviewers for their comments that improved this manuscript.
Literature Cited
Beyer, H.L. 2015. Geospatial Modelling Environment (Version 0.7.4.0). (software). Available
online at http://www.spatialecology.com/gme. Accessed 22 November 2016.
Burnham, K.P., and D.R. Anderson. 1998. Model Selection and Inference: A Practical
Information-Theoretic Approach. Springer-Verlag, New York, NY. 353 pp.
Calcagno, V. 2015. Package ‘glmulti’. (software). Available online at https://cran.r-project.
org/web/packages/glmulti/glmulti.pdf. Accessed 15 May 2017.
Calcagno, V., and C. de Mazancourt. 2010. glmulti: An R package for easy automated
model-selection with (generalized) linear models. Journal of Statistical Software.
34:12. Available online at https://www.jstatsoft.org/article/view/v034i12. Accessed 15
May 2017.
Chase, J.D., W.E. Howard, and J.T. Rosenberry. 1982. Pocket gophers, Geomyidae. Pp.
239–255, In J.A. Chapman and G.A. Feldhamer (Eds.). Wild Mammals of North America:
Biology, Management, and Economics. Johns Hopkins University Press, Baltimore,
MD. 1168 pp.
Connior, M.B. 2011. Histeridae and Scarabaeidae from unsampled populations of pocket
gopher burrows in Louisiana. Insecta Mundi 153:1–5.
Connior, M.B., and T.S. Risch. 2009. Live trap for pocket gophers. Southwestern Naturalist
54:100–103.
Connior, M.B., and T.S. Risch. 2010. Home range and survival of the Ozark Pocket Gopher
(Geomys bursarius ozarkensis) in Arkansas. American Midland Naturalist 164:80–90.
Connior, M.B., R. Tumlison, and H.W. Robison. 2011. New records and notes on the natural
history of selected vertebrates of Arkansas. Journal of the Arkansas Academy of Science
65:160–165.
Connior, M.B., R. Tumlison, H.W. Robison, C.T. McAllister, and D.A. Neely. 2014. Natural
history notes and records of vertebrates from Arkansas. Journal of the Arkansas Academy
of Science 68:140–145.
Southeastern Naturalist
409
R.O. Wagner, M.B. Connior, C.A. Melder, B.S. Cooper, D. Hightower, and S. Pearce
2017 Vol. 16, No. 3
Davis, W.B., R.R. Ramsey, and J.M. Arendale Jr. 1938. Distribution of pocket gophers
(Geomys breviceps) in relation to soils. Journal of Mammalogy 66:489–497.
Gannon, W.L., R.S. Sikes, and the Animal Care and Use Committee of the American Society
of Mammalogists. 2007. Guidelines of the American Society of Mammalogists for
the use of wild mammals in research. Journal of Mammalogy 88:809–823.
Harrell, F.E., Jr. 2001. Regression Modeling Strategies with Applications to Linear Models,
Logistic Regression, and Survival Analysis. Springer-Verlag, New York, NY. 568 pp.
Harrell, F.E., Jr. 2017. Package ‘rms’. (software). Online at https://cran.r-project.org/web/
packages/rms/rms.pdf. Accessed 15 May 2017.
Himes, J.G., L.M. Hardy, D.C. Rudolph, and S.J. Burgdorf. 2006. Movement patterns and
habitat selection by native and repatriated Louisiana Pine Snakes (Pituophis ruthveni):
Implications for conservation. Herpetological Natural History 9:103–116.
Huntly, N., and R. Inouye. 1988. Pocket gophers in ecosystems: Patterns and mechanisms.
Bioscience 38:786–793.
Johnson, C.J., S.E. Nielsen, E.H. Merrill, T.L. McDonald, and M.S. Boyce. 2006. Resource
selection functions based on use-availability data: Theoretical motivation and evaluation
methods. Journal of Wildlife Management 70:347–357.
King, K.N. 2010. Site fidelity and population structure of a solitary subterranean rodent,
Geomys breviceps, in southwestern Arkansas. M.Sc. Thesis. University of Arkansas,
Little Rock, AR. 42 pp.
Lemmon, P.E. 1957. A new instrument for measuring forest overstory density. Journal of
Forestry 55:667–669.
Lowery, G.H. 1974. Mammals of Louisiana and Its Adjacent Waters. Louisiana State University
Press, Baton Rouge, LA. 565 pp.
Luce, D.G., R.M. Case, J. Stubbendieck. 1980. Food habits of the Plains Pocket Gopher on
western Nebraska rangeland. Journal of Range Management 33(2):129–131.
Manly, B.F.J, L.L. McDonald, D.L. Thomas, T.L. McDonald, and W.P. Erickson. 2002.
Resource Selection by Animals, Statistical Design and Analysis for Field Studies. 2nd
Edition. Kluwer Academic Publishers, Dordrecht, The Netherlands. 221pp.
Myers, G.T, and T.A. Vaughan. 1964. Food habits of the Plains Pocket Gopher in eastern
Colorado. Journal of Mammalogy 45:588–598.
R Development Core Team. 2016. R: A Language and Environment for Statistical Computing.
R Foundation for Statistical Computing, Vienna, Austria. (software). Available
online at http://www.R-project.org/. Accessed 22 November 2016.
Reichman, O.J., and E.W. Seabloom. 2002. The role of pocket gophers as subterranean
ecosystem engineers. Trends in Ecology and Evolution 17(1):44–49.
Rudolph, D.C., S.J. Burgdorf, R.N. Conner, C.S. Collins, D. Saenz, R.R. Schaefer, and T.
Trees. 2002. Prey handling and diet of the Louisiana Pine Snake (Pituophis ruthveni)
and Black Pine Snake (P. melanoleucus lodingi), with comparisons to other selected
colubrid taxa. Herpetological Natural History 9:57–62.
Rudolph, D.C., C.A. Melder, J. Pierce, R.R. Schaefer, and B. Gregory. 2012. Diet of the
Louisiana Pine Snake (Pituophis ruthveni). Herpetological Review 43:243–245.
Samuel, M.D., and M.R. Fuller. 1994. Wildlife radiotelemetry. Pp. 370–418, In T.A. Bookhout
(Ed). Research and Management Techniques for Wildlife and Habitats. 5th Edition.
The Wildlife Society, Bethesda, MD. 740 pp.
Sulentich, J.M., L.R. Williams, and G.N. Cameron. 1991. Geomys breviceps. Mammalian
Species 383:1–4.
US Fish and Wildlife Service (USFWS). 2003. Recovery plan for the Red-cockaded Woodpecker
(Picoides borealis): Second revision. Atlanta, GA. 296 pp.
Southeastern Naturalist
R.O. Wagner, M.B. Connior, C.A. Melder, B.S. Cooper, D. Hightower, and S. Pearce
2017 Vol. 16, No. 3
410
US Geological Survey (USGS). 2016. National Elevation Dataset. Washington, DC.
Available online at https://lta.cr.usgs.gov/NED. Accessed 18 November 2016.
Vleck, D. 1979. The energy cost of burrowing by the pocket gopher Thomomys bottae.
Physiological Zoology 52:122–136.
Wagner, R.O., J.B. Pierce, D.C. Rudolph, R.R. Schaefer, and D.A. Hightower. 2014. Modeling
Louisiana Pine Snake (Pituophis ruthveni) habitat use in relation to soils. Southeastern
Naturalist. 13(Special Issue 5):146–158.
Warren, A.E. 2014. Ecology of the Southeastern Pocket Gopher (Geomys pinetis) in southwestern
Georgia. M.Sc. Thesis. University of Georgia, Athens, GA. 79 pp.
Wilkins, K.T., and H.R. Roberts. 2007. Comparative analysis of burrow systems of seven
species of pocket gophers (Rodentia: Geomyidae). Southwestern Naturalist 52:83–88.
Wilks, B.J. 1963. Some aspects of the ecology and population dynamics of the pocket gopher
(Geomys bursarius) in southern Texas. Texas Journal of Science 15:241–283.