Ozone Sensitivity of 28 Plant Selections Exposed to Ozone
Under Controlled Conditions
Lee J. Kline, Donald D. Davis, John M. Skelly, James E. Savage,
and Jon Ferdinand
Northeastern Naturalist, Volume 15, Issue 1 (2008): 57–66
Full-text pdf (Accessible only to subscribers.To subscribe click here.)

Access Journal Content
Open access browsing of table of contents and abstract pages. Full text pdfs available for download for subscribers.
Current Issue: Vol. 30 (3)
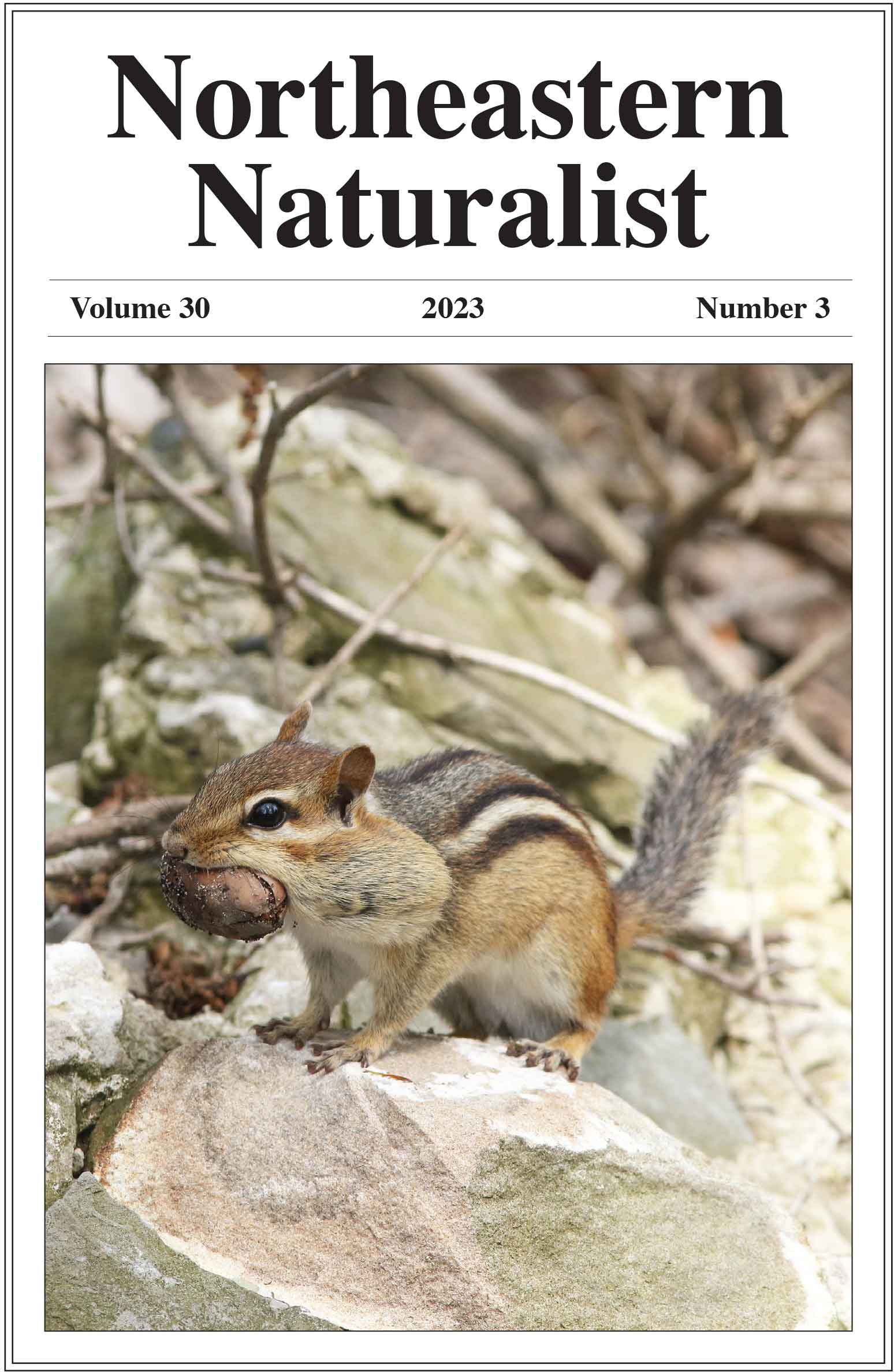
Check out NENA's latest Monograph:
Monograph 22
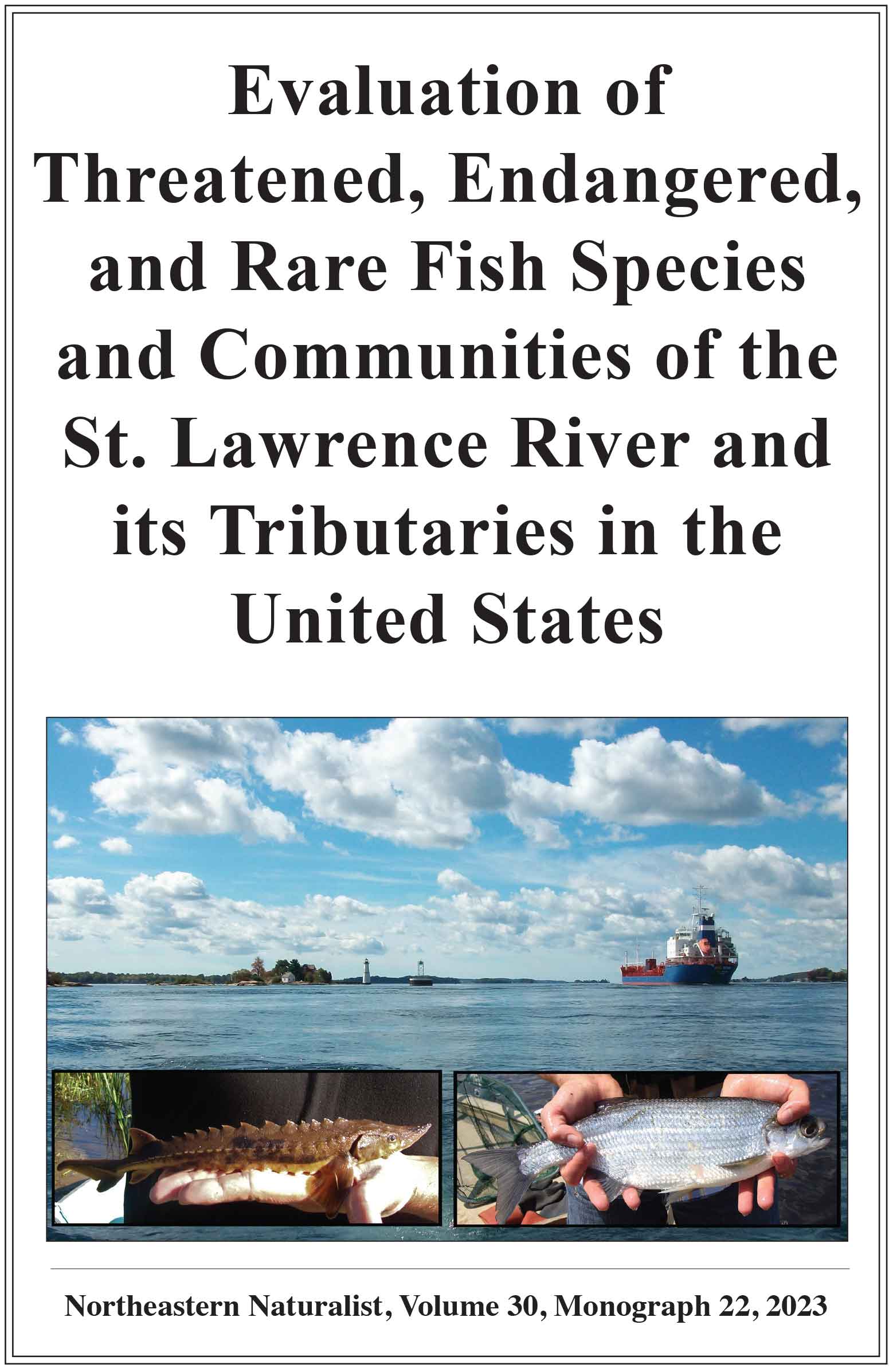
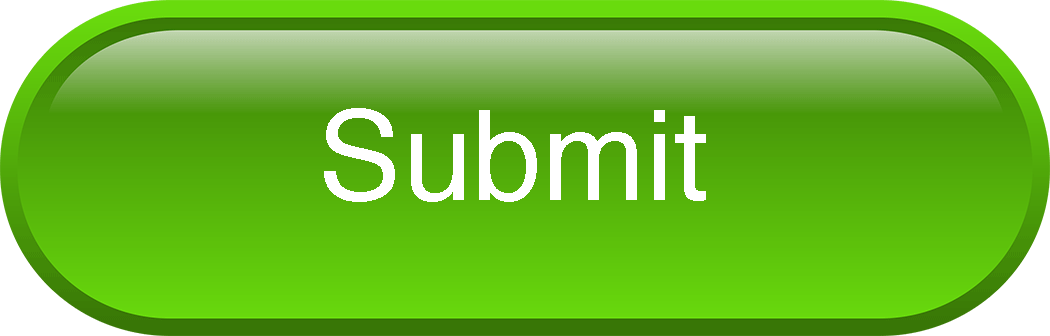
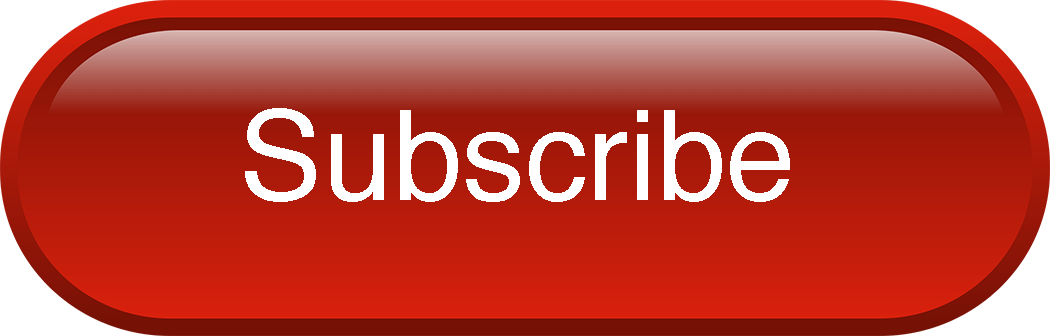
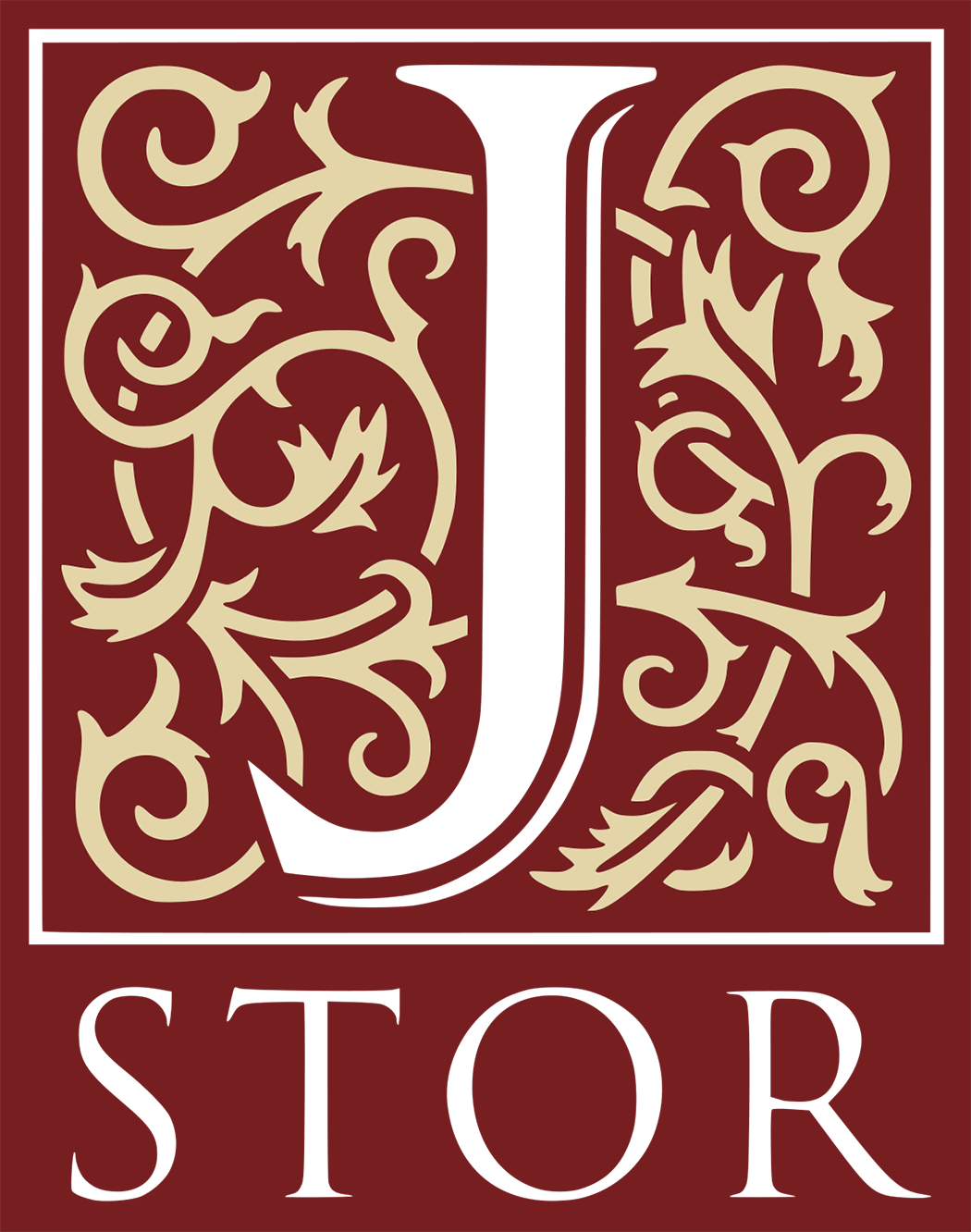
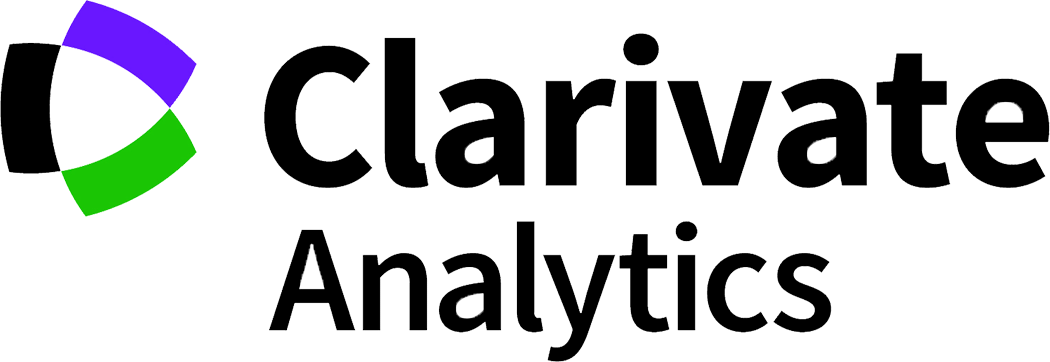
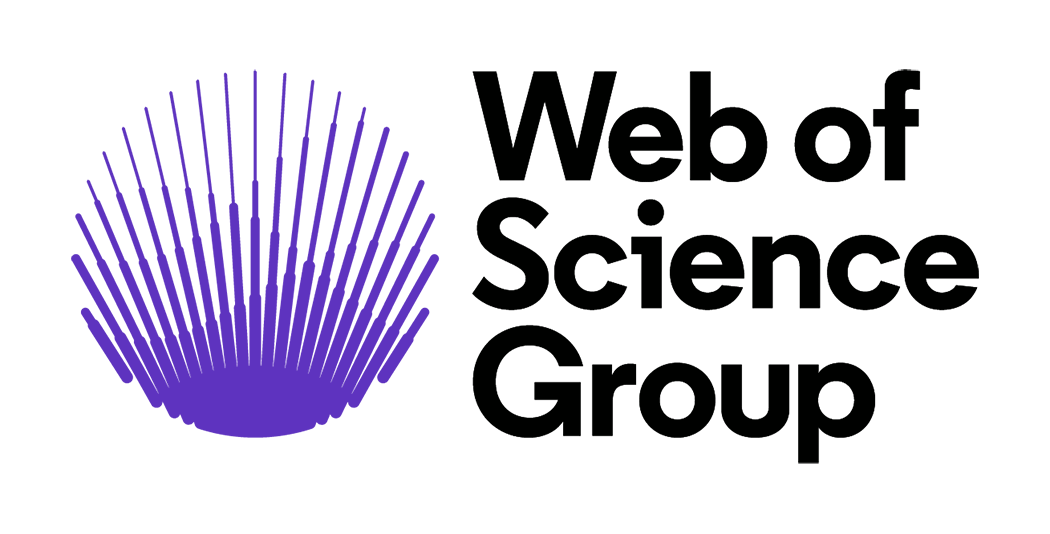
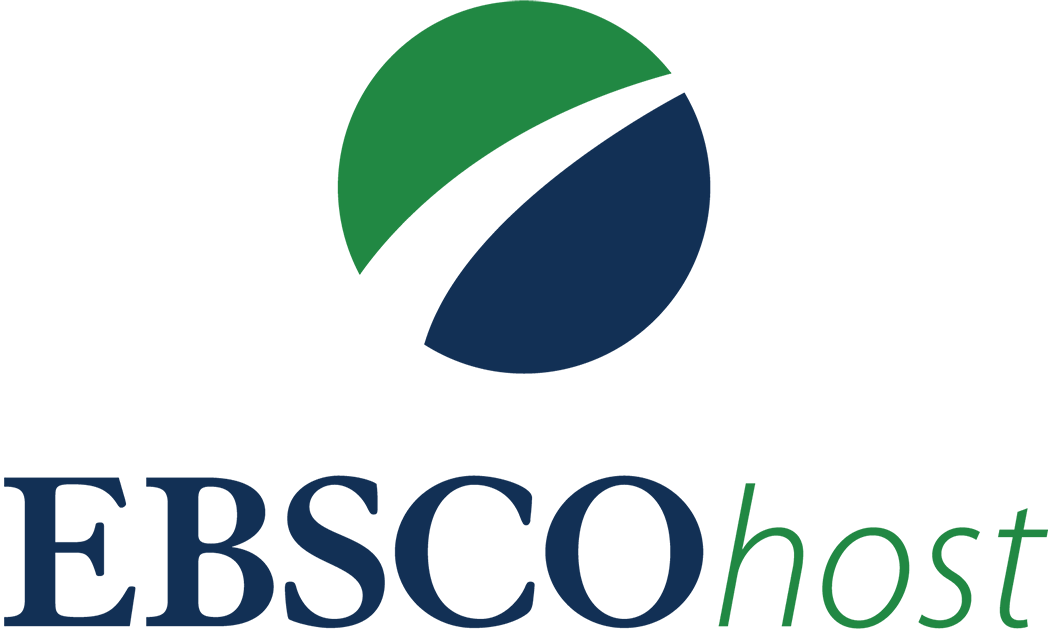
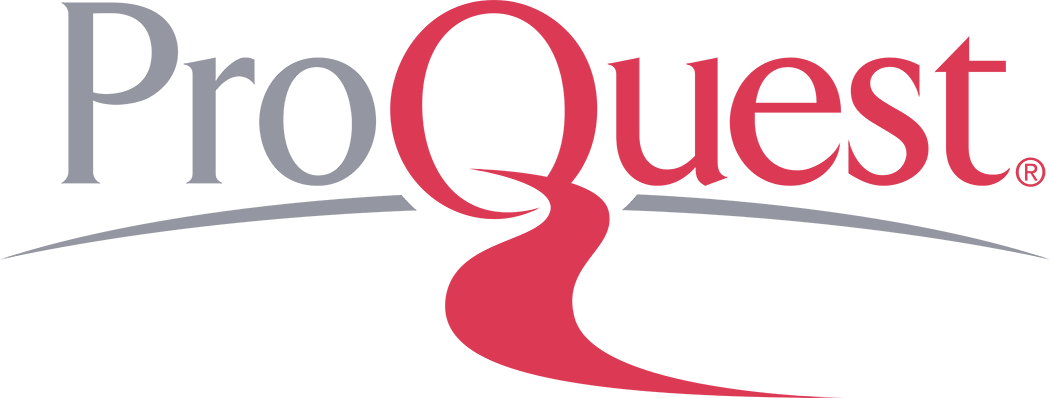
2008 NORTHEASTERN NATURALIST 15(1):57–66
Ozone Sensitivity of 28 Plant Selections Exposed to Ozone
Under Controlled Conditions
Lee J. Kline1, Donald D. Davis1,*, John M. Skelly1, James E. Savage1,
and Jon Ferdinand1
Abstract - Ambient, ground-level ozone is the most important air pollutant affecting
vegetation in the US. However, ozone-sensitive bioindicators need to be
identified for use in field surveys to detect ozone-induced symptoms. To identify
such bioindicators, 28 plant selections were exposed to ozone within greenhouse
chambers during 2003 and 2004. Plants most sensitive to ozone included Asclepias
incarnata (swamp milkweed), Asclepias syriaca (common milkweed), Cephalanthus
occidentalis (buttonbush), Platanus occidentalis (American sycamore), Salix
x cotteti (Bankers dwarf willow), Salix lucida (shining willow), Salix nigra (black
willow), Salix sericea (silky willow), and Symphoricarpos albus (snowberry).
Plants moderately sensitive included Aster novae-angliae (New England aster),
Monarda didyma (bee-balm), Rhus aromatica (aromatic sumac), Salix discolor
(pussy willow), Salix exigua (sandbar willow), Salix purpurea (basket willow),
Sambucus ebulus (European dwarf elderberry), and Symphoricarpos spp. (mixture
of “snowberries”). Plants more tolerant to ozone included Aster macrophyllus
(bigleaf aster), Aster novi-belgii (New York aster), Cercis canadensis (redbud),
Populus maximowizii x trichocarpa (hybrid poplar), Rudbeckia laciniata (cutleaf,
or tall green-headed coneflower), Salix amygdaloides (peach-leaved willow), Salix
eriocephala (diamond willow), Sambucus canadensis (American elder), Sambucus
nigra (European elder), Symphoricarpos orbiculatus (coralberry), and Viburnum
trilobum (highbush-cranberry). The incidence and severity of ozone-induced symptoms
varied with species, ozone concentration, and length of exposure. Pigmented
adaxial leaf surface stipple, bifacial necrosis, and premature defoliation were common
foliar symptoms induced by ozone; leaf reddening, bronzing, and chlorosis
occurred less frequently. Species that produced classic ozone-induced stipple in
this study may serve as useful bioindicators in field surveys to confirm that ozone
injury occurs in many parts of the country. Such findings may have a positive impact
on establishing more stringent air-quality standards to protect vegetation and
the environment.
Introduction
Ambient, ground-level ozone is the most important air pollutant affecting
vegetation in the eastern US (Skelly 2000). Many universities
and governmental organizations perform field surveys to determine if
ozone is adversely impacting vegetation in remote areas, but lack suitable
ozone-sensitive bioindicators for use in many parts of the country.
1Department of Plant Pathology and Penn State Institutes of the Environment and
Energy, The Pennsylvania State University, University Park, PA 16802. *Corresponding
author - ddd2@psu.edu.
58 Northeastern Naturalist Vol. 15, No. 1
The objective of such surveys is to document the incidence or severity
of ozone-induced symptoms in relatively pristine areas, with the hope
that results will be used in establishing more stringent ambient air-quality
standards for ozone (US Congress 1977, US EPA 1996). Such surveys
often utilize ozone-sensitive, broadleaved bioindicator plants (Coulston et
al. 2003, US DOI 2003) that respond to ambient ozone by exhibiting foliar
“stipple” (Richards et al. 1958), a characteristic symptom induced by
excessive ambient ozone (Skelly 2000). During field surveys, crews often
report ambiguous symptoms observed on additional broadleaved species.
To confirm species sensitivity and to describe ozone-induced symptoms,
these additional species must be exposed to ozone under controlled conditions,
such as in continuously stirred tank reactor (CSTR) chambers
(Heck et al. 1975). The objectives of this study were to evaluate the ozone
sensitivity of 28 plant selections and to describe ozone-induced symptoms
produced under controlled conditions.
Methods
Plant culture and exposure to ozone
Most plant selections chosen for this study (Table 1) grow naturally
throughout the midwest and northeast USA. Selections were suspected to
be sensitive to ozone based on field symptoms, were within genera known
to be ozone-sensitive (Innes et al. 2001, Skelly et al. 2000, VanderHeyden
et al. 2000), or were fast-growing, intolerant plants. Fast-growing species
are often sensitive to air pollutants (Harkov and Brennan 1979, Umbach
and Davis 1984). Milkweed plants were grown from seed. Elderberry,
poplar, and willow selections were grown from vegetative cuttings;
remaining selections were obtained as bare-root seedlings. Plants were
potted in Metromix 500® potting soil (Scotts-Sierra Horticultural Products
Co., Marysville, OH) supplemented with a one-time application of
5 g Osmocote® controlled-release fertilizer (Scotts-Sierra Horticultural
Products Co.) at time of potting.
Potted plants were maintained on benches in a greenhouse supplied
with charcoal-filtered air (less than 8 ppb ozone daily hourly average) until placement
into CSTR chambers for exposure to ozone. Plants were routinely
watered prior to, during, and after exposures. Four ozone concentrations
(30, 60, 90, and 120 ppb) were generated in a square-wave fashion for 7
hrs/day, 5 days/week within the chambers. Experiments began at 0900 hrs
and ended at 1600 hrs daily. The ozone level of 30 ppb was intended to
approximate background ozone concentrations, 60 ppb reflected a typical
daily 7–8 hr ambient ozone exposure during mid- to late-summer in
central Pennsylvania, and 90 ppb was employed to induce symptoms on
less sensitive species (Orendovici et al. 2003). Lastly, the 120 ppb concentration
was selected to induce foliar symptoms on the least sensitive
species. During non-exposure hours, plants remained in the chambers
with the chamber doors open and were exposed to the charcoal-filtered
2008 L.J. Kline, D.D. Davis, J.M. Skelly, J.E. Savage, and J. Ferdinand 59
air and environmental conditions within the greenhouse. Control plants
were maintained on greenhouse benches, outside of the CSTR chambers,
and received charcoal-filtered air.
In 2003, 12 plant species (Table 1) were used in two consecutive studies.
Ozone exposures during the first study started on 14 July and ended
Table 1. Ozone sensitivity of plants exposed in 2003 and 2004, based on a combined rating of
percent symptomatic leaf tissue and severity of symptoms for each species. Average injury is the
row mean calculated across the 30, 60, 90, and 120 ppb response for each species.
# of %INJ by ozone level (ppb) Average
Year/species plants 30 60 90 120 injuryA
2003
Platanus occidentalis L. (American sycamore) 8 0.00 0.06 17.00 44.44 15.38 a
Asclepias syriaca L. (common milkweed) 8 0.00 0.00 16.78 33.44 12.56 ab
Asclepias incarnata L. (swamp milkweed) 4 0.00 0.00 14.44 33.44 11.97 ab
Symphoricarpos albus (L.) S.F. Blake 16 0.06 0.17 7.10 14.95 7.43 ab
(snowberry)
Rhus aromatica Aiton (aromatic sumac) 24 0.00 0.06 4.55 18.53 5.79 ab
Symphoricarpos spp. (mixed snowberries) 44 0.01 0.77 1.48 13.67 3.98 ab
Sambucus nigra L. (European elder) 24 0.02 0.04 0.10 6.43 1.65 b
Sambucus canadensis L. (American elder) 28 0.00 0.02 1.33 4.48 1.46 b
Cercis canadensis L. (redbud) 8 0.00 0.00 1.28 1.56 0.71 b
Symphoricarpos orbiculatus Moench 20 0.00 0.00 0.21 1.23 0.24 b
(coralberry)
Viburnum trilobum Marshall 8 0.00 0.00 0.06 0.34 0.10 b
(highbush-cranberry)
Aster macrophyllus L. (bigleaf aster) 8 0.00 0.00 0.06 0.34 0.10 b
2004
Salix nigra Marshall (black willow) 12 B B 17.07 33.94 25.50C a
Salix sericea Marshall (silky willow) 22 0.02 12.80 16.85 49.14 19.26 ab
Salix lucida Muhl. (shining willow) 23 0.00 17.61 17.22 34.32 18.04 bc
Salix x cotteti (Bankers dwarf willow) 24 0.00 2.83 25.42 40.73 17.24 bcd
Cephalanthus occidentalis L. (buttonbush) 16 0.06 4.71 13.25 49.12 16.78 bcd
Salix exigua Nutt. (sandbar willow) 24 3.38 8.93 11.11 31.81 13.81 bcde
Salix purpurea L. (basket willow) 24 0.00 0.00 12.74 33.44 11.54 bcdef
Aster novae-angliae L. 24 0.02 0.95 16.28 28.22 11.38 cdef
(New England aster)
Sambucus ebulus L. 19 0.02 6.50 18.15 15.04 9.89 def
(European dwarf elderberry)
Salix discolor Muhl. (pussy willow) 23 0.00 3.70 7.62 21.21 8.48 efg
Monarda didyma L. (bee-balm) 18 0.04 1.77 9.30 16.04 6.78 efg
Aster novi-belgii L. (New York aster) 24 0.08 2.67 2.07 21.51 6.58 efg
Salix eriocephala Michx (diamond willow) 24 0.04 1.27 5.16 13.17 4.87 fg
Populus maximowizii x trichocarpa 20 0.60 4.18 5.07 6.90 4.18 fg
(hybrid poplar)
Rudbeckia laciniata L. (cutleaf coneflower) 20 0.00 0.60 2.02 12.00 3.80 fg
Salix amygdaloides Andersson 20 0.00 0.09 0.34 2.16 0.71 g
(peach-leaved willow)
AMeans in the final column (average injury) followed by the same letter are not sigificantly different.
(p = 0.05) within that year, according to Duncan’s multiple range test.
BBlank space indicates no data.
CMean based only on 90 and 120 ppb data.
60 Northeastern Naturalist Vol. 15, No. 1
on 21 August. There were three replications (three chambers) for each
ozone level (four concentrations), using 12 CSTR chambers. Exposures in
the second study started on 9 September and ended on 30 September. Due
to mechanical difficulties, only four CSTR chambers were utilized in the
second 2003 exposure, involving one chamber for each level of ozone. In
both studies, the number of individual plants varied slightly depending
upon plant condition, but usually involved two plants/species/chamber. In
2004, 16 plant selections, including two hybrids (Table 1), were exposed
during two consecutive studies, using methods generally as described
above. In the first 2004 study, ozone exposures were conducted in eight
chambers from 13 July to 10 August. In the second study, plants were exposed
in 12 chambers from 27 August to 24 September.
Ozone concentration, light, relative humidity, and temperature were
monitored within each chamber for 1.5 min at 12-min intervals during
all exposures. Ozone was sampled through Teflon tubing using a solenoid-
driven sampling system connected to a TECO Model 49 photometric
ozone analyzer (Thermo Environmental Corp., Franklin, MA). Ozone
calibration quality-control measures for the TECO analyzer followed the
standards documented by the Pennsylvania Department of Environmental
Protection, Bureau of Air Quality, Harrisburg, PA. Ozone and environmental
data were input to a data logger connected to a computer.
Data collection and analyses
Each plant was rated for amount of foliage injured (AMT: % leaves
symptomatic) and the severity of the injured foliage (SEV: % area affected
on symptomatic leaves) as described by Orendovici et al. (2003).
The assessments estimated the percentage of symptomatic tissue and were
assigned nominal values that reflect five broad classes: 0 = no symptoms,
1 = 1–6%, 2 = 7–25%, 3 = 26–50%, 4 = 51–75%, and 5 = 75–100% tissue
symptomatic. These data were used to calculate an overall value for
each plant, as well as a mean value for each species. The nominal values
recorded for each plant were converted to percentage values representing
the midpoint of each class as follows: 0 = 0%, 1 = 3.5%, 2 = 16%, 3 =
38%, 4 = 63%, and 5 = 88%. Percentage values were calculated per plant
and per species: mean injury value (%INJp) per plant = AMT*SEV, and
mean injury value (%INJs) per species = (AMT*SEV)/N, where N is the
number of plants evaluated per species. The statistical design was a split
plot, with ozone treatments as the main plot and species as the subplot. To
increase the number of observations for statistical analyses, data from the
two studies in each year were combined. A general linear model (GLM)
was utilized to analyze the percentage data (%INJs) and means were separated
using Duncan’s multiple range test (Minitab 2003). Control plants
did not exhibit any symptoms similar to those induced by ozone on the
plants exposed within the CSTR chambers.
2008 L.J. Kline, D.D. Davis, J.M. Skelly, J.E. Savage, and J. Ferdinand 61
Results
Ozone and environmental monitoring
2003. In the first study, the mean ozone concentrations achieved in the
chambers for target concentrations of 30, 60, 90, and 120 ppb were 27.9,
56.6, 84.3, and 113.8 ppb, respectively. Mean ozone concentrations for
the four target levels in the second study were 26.3, 55.8, 83.3, and 113.8
ppb. Average temperatures monitored within the chambers in the two
studies were 27.5 and 21.5 °C, and mean relative humidities were 84 and
83%, respectively.
2004. In the first study, the respective mean ozone concentrations for
targets of 30, 60, 90, and 120 ppb were 27.1, 57.9, 87.3, and 117.4 ppb, respectively.
In the second study, the respective monitored ozone levels were
29.3, 59.0, 87.8, and 119.7 ppb. Temperatures within the exposure chambers
in the two studies were 26.2 and 28.3 °C, and mean relative humidities were
84 and 77%, respectively.
Foliar symptoms
2003. Adaxial leaf-surface stippling, the classic foliar response of
broadleaved plants to ozone (Richards et al. 1958, Skelly 2000), was
observed on sensitive plant selections. Stipple progressed from light
to dark in color with increasing length of exposure or increasing ozone
concentration. Classic dark stipple was most evident on the more mature
leaves that were present later in the exposures. Premature defoliation was
also noted on sensitive plants. Ozone induced other occasional symptoms
including tan stipple, chlorotic or necrotic spotting, general chlorosis, and
reddening, but these symptoms were not rated.
Plant species that exhibited classic, dark stipple in response to ozone
included American elder, American sycamore, aromatic sumac, common
milkweed, highbush-cranberry, redbud, and snowberry. On swamp
milkweed, the stipple was initially tan, but became darker as exposures
progressed. Common symptoms on coralberry included general chlorosis,
followed by the classic dark stipple that intensified with increasing exposure.
The predominant leaf symptom on bigleaf aster was a grayish-white
stipple that was fine and “grainy” in appearance. European elder consistently
exhibited a white bifacial fleck.
Foliage of species with high levels of red leaf pigments has a tendency
to develop a reddish color upon exposure to ozone (Orendovici et al.
2003). Aromatic sumac most consistently exhibited foliar reddening, in
addition to stipple, at higher exposures.
2004. Adaxial leaf-surface stippling was observed on several species.
As in 2003, stipple often was initially light-colored, and became darker
with increasing ozone. Other ozone-induced symptoms were occasionally
observed, including tan stipple, chlorotic spotting, chlorosis, reddening,
bronzing, necrosis, and premature defoliation.
62 Northeastern Naturalist Vol. 15, No. 1
Plant species that most commonly developed the classic dark stipple
were Bankers dwarf willow, bee-balm, and occasionally cutleaf coneflower.
In addition, coneflower exhibited a dark-gray to dark-brown, irregular discoloration
on upper leaf surfaces, and buttonbush exhibited a tan stipple that
became darker with length of exposure time. A whitish-tan stipple occurred
on the upper leaf surfaces of basket willow, European dwarf elderberry,
peach-leaved willow, and silky willow.
Foliar reddening occurred on Bankers dwarf willow and buttonbush
at greater ozone exposures. Ozone-induced symptoms on most species of
willows appeared as orange, tan, or rust-colored angular spots, usually on
the edges or tips of leaves. Other symptoms on willow included tip burn,
bifacial edge necrosis, chlorosis, and premature defoliation. Black willow,
pussy willow, and silky willow exhibited the most severe symptoms of the
willows. Foliage of New England aster and New York aster slowly faded in
luster and color during exposure, until leaves eventually became light tan
and then entirely necrotic. Large areas of black bifacial necrosis and premature
defoliation occurred on hybrid poplar. Chlorosis was evident to some
degree on the foliage of most sensitive species.
Response to different levels of ozone
2003. Following exposure to 30 ppb, the lowest ozone concentration
utilized, a few individual European elder, snowberry, and the mixture of
snowberries exhibited only traces of ozone-induced symptoms (Table 1).
At 60 ppb, these species plus American elder, American sycamore, and
aromatic sumac developed symptoms. All species exhibited some symptoms
at 90 ppb ozone. American sycamore, common milkweed, and
swamp milkweed were clearly symptomatic at 90 ppb, but bigleaf aster,
redbud, European elder, and highbush-cranberry were only slightly
symptomatic at this concentration. At 120 ppb ozone, some individuals
within all selections exhibited symptoms. American sycamore, common
milkweed, and swamp milkweed exhibited considerable foliar symptoms,
but approximately half of the selections were somewhat insensitive to 120
ppb, showing only light symptoms. Bigleaf aster and highbush-cranberry
were least sensitive. Exposure to highter concentrations of ozone generally
resulted in increased symptoms on most species.
2004. There was only a trace of foliar symptoms following exposure
to 30 ppb ozone (Table 1). An exception was shining willow, which consistently
exhibited rust-colored angular spots, usually on the edges or tips
of leaves, following exposure to 30 ppb. All species except basket willow
showed symptoms at 60 ppb, although symptoms on peach-leaved
willow were extremely light. Due to the low number of plants available,
black willow was only exposed to 90 and 120 ppb. Most species were
clearly symptomatic following exposure to 90 and 120 ppb ozone, but
symptoms on peach-leaved willow were very light.
2008 L.J. Kline, D.D. Davis, J.M. Skelly, J.E. Savage, and J. Ferdinand 63
Ranking of species sensitivity
2003. American sycamore was most sensitive to ozone, followed in
descending order by common milkweed, swamp milkweed, and snowberry
(Table 1). The level of symptoms on these four species was similar (p =
0.05). Aromatic sumac, followed by mixed snowberries, European elder, and
American elder, ranked next and were generally similar in ozone-sensitivity.
Redbud was somewhat insensitive to ozone. Coralberry, bigleaf aster, and
highbush-cranberry were least sensitive. However, the mean response values
of the last nine selections (Table 1) were not significantly different, likely
due to the high variability in the data.
2004. Among the willows, black willow was very sensitive to ozone
(Table 1). However, due to the small number of available plants, black willow
was only exposed at the two greater levels of ozone, resulting in inflated
response values. Nevertheless, if one compares the symptom values for this
species following exposure to 90 and 120 ppb with that induced on other
species, black willow still ranks among the more sensitive willows. Shining
willow, silky willow, and Bankers dwarf willow were also quite sensitive to
ozone, as were sandbar willow and basket willow. Pussy willow showed few
ozone-induced symptoms, diamond willow exhibited fewer symptoms, and
peach-leaved willow was insensitive. Among plants other than willows, buttonbush
was most sensitive, followed by New England aster and European
dwarf elderberry. Bee-balm, cutleaf coneflower, New York aster, and hybrid
poplar were less sensitive.
Discussion
The main objectives of this study were to evaluate the relative ozone
sensitivity of different plant selections and to describe ozone-induced foliar
symptoms under controlled conditions in CSTR chambers. The most
sensitive plants included American sycamore, aromatic sumac, basket willow,
Bankers dwarf willow, bee-balm, black willow, buttonbush, common
milkweed, European dwarf elderberry, New England aster, sandbar willow,
shining willow, silky willow, snowberry, and swamp milkweed. These species
are potential bioindicators for use in ozone surveys. However, ozone
caused marginal and tip leafburn on the willows, symptoms that would be
very difficult to attribute to ozone in the field. Therefore, we propose that
the most useful bioindicators are American sycamore, aromatic sumac, beebalm,
buttonbush, common milkweed, European dwarf elderberry, New
England aster, snowberry, and swamp milkweed.
Our study confirmed earlier reports that the following species were sensitive
to ozone: swamp milkweed (Orendovici et al. 2003), common milkweed
(Duchelle and Skelly 1981), American sycamore (Davis and Coppolino
1976, Neufeld and Renfro 1993), European dwarf elderberry (Orendovici
et al. 2003), and snowberry (Davis and Coppolino 1976). We previously
reported hybrid poplar to be sensitive to ozone when exposed within growth
chambers in a laboratory (Davis et al. 1993), but the same clone was rather
64 Northeastern Naturalist Vol. 15, No. 1
insensitive in the study reported herein. This discrepancy was likely due
to different exposure/environmental conditions between the two studies.
Cutleaf coneflower is very sensitive to ambient ozone in the field (Chappelka
et al. 2003), but our selection was quite tolerant. Our coneflower seed
was collected from the field in southeastern Pennsylvania, within an area
that has very high levels of ambient ozone. Coneflower populations that
exhibited ozone-induced symptoms, such as discolored leaves and reduced
growth, were likely not collected, and thus, ozone-tolerant populations of
cutleaf coneflower may have been inadvertently selected by seed collectors.
Genetic variation in sensitivity among different populations of plants, as
well as intraspecific variability in ozone sensitivity among individual plants
within the same species, is well known (Karnosky et al. 2005). In our study,
this high degree of variability often limited statistical analysis when testing
for within-species differences in sensitivity. Thus, the rankings in this paper
must be considered only as a general guide.
Sensitive species other than willows often exhibited classic adaxial
leaf surface stippling as the predominant symptom following ozone exposure.
Stipple was first described as being a symptom of ozone response by
Richards et al. (1958) on grapes and has since been defined as the classic
symptom of ozone-induced symptoms on broadleaved species (Skelly 2000).
Ozone-induced stipple, as observed in these CSTR studies, was often similar
to foliar symptoms attributed to ambient ozone in the field. The broadleaved
species that produced classic stipple in this study may serve as useful bioindicators.
However, nearly half of the sensitive selections exhibited at least
one non-specific symptom, such as foliar reddening, premature defoliation,
bronzing, flecking, bleaching, leaf-tip burn, chlorosis, necrosis, and spotting.
The bifacial fleck that we observed on European elder was similar to
that reported by VanderHeyden et al. (2000) during field studies in Switzerland.
Such symptoms are not reliable tools when assessing ozone-induced
symptoms, since they could be caused by biotic and abiotic factors other than
ozone (Orendovici et al. 2003). Species that routinely exhibit non-specific
symptoms in response to ozone may not be useful bioindicators. However,
induction of such symptoms indicates that ozone may be causing adverse
symptoms on vegetation that have not previously been recognized as being
induced by ozone.
Many plant species in this study were selected for their potentially high
sensitivity to ozone (US DOI 2003). Thus, even the “insensitive” species as
defined by this study may be symptomatic in the field, since they often developed
symptoms following exposure to 60 ppb ozone, below the proposed
national ambient air quality standard. However, extrapolation of these CSTR
results to the field must be done carefully, since CSTR/greenhouse conditions
may influence ozone response and are not representative of natural
environmental conditions. Additional studies are needed wherein potential
bioindicator species are exposed to ozone in the field under more realistic
conditions, such as in open-top chambers. Nevertheless, across much
2008 L.J. Kline, D.D. Davis, J.M. Skelly, J.E. Savage, and J. Ferdinand 65
of the US, phytotoxic levels of ozone occur during each growing season,
and ozone-induced injury to native vegetation in these areas will likely
continue in future years. Sensitive bioindicators are useful tools to confirm
the occurrence of ozone injury in many parts of the country, including areas
previously thought to be “pristine.” Such findings may have a positive
impact on establishing more stringent air-quality standards to protect vegetation
and the environment, ultimately resulting in cleaner air.
Acknowledgments
The authors acknowledge financial support from the USDA Forest Service and
the University of Massachusetts, and technical assistance from T. Orendovici-Best.
The authors also thank the USDA Forest Service and their cooperators for supplying
plant material.
Literature Cited
Chappelka, A., H. Neufeld, A. Davison, G. Somers, and J. Renfro. 2003. Ozone
injury on cutleaf coneflower (Rudbeckia laciniata) and crown-beard (Verbesina
occidentalis) in Great Smoky Mountains National Park. Environmental
Pollution 125:53–59.
Coulston, J.W., G.C. Smith, and W.D. Smith. 2003. Regional assessment of
ozone-sensitive tree species using bioindicator plants. Environmental Monitoring
and Assessment 83:117–127.
Davis, D.D., and J.B. Coppolino. 1976. Ozone susceptibility of selected woody
shrubs and vines. Plant Disease Reporter 60:876–878.
Davis, D.D., R.J. Hutnik, and J.R. McClenahen. 1993. Evaluation of vegetation
near coal-burning power plants in southwestern Pennsylvania. Journal of Air
Waste Management Association 43:760–764.
Duchelle, S.F., and J.M. Skelly. 1981. Response of common milkweed to oxidant
air pollution in the Shenandoah National Park in Virginia. Plant Disease 65:
661–663.
Harkov, R., and E. Brennan. 1979. An ecophysiological analysis of the response
of trees to oxidant pollution. Journal of Air Pollution Control Association 29:
157–161.
Heck, W.W., R.B. Philbeck, and J.A. Dunning. 1975. A continuous stirred tank reactor
(CSTR) System for exposing plants to gaseous contaminants: Principles, specifications,
construction, and operation. USDA-ARS Pub. No. ARS-S-181. US Department
of Agriculture, Agricultural Research Service, New Orleans, LA.
Innes, J.L., J.M. Skelly, and M. Schaub. 2001. A Guide to the Identification of
Ozone-induced Foliar Injury on Broadleaved Tree, Shrub, and Herb Species.
Paul Haupt Publishing, Bern, Switzerland. 136 pp.
Karnosky, D.F., K.S. Pregitzer, D.R. Zak, M.E. Kubiske, G.R. Hendrey, D. Weinstein,
M. Nosal, and K.E. Percy. 2005. Scaling ozone responses of forest trees
to the ecosystem level in a changing climate. Plant, Cell, and Environment 28:
965–981.
Minitab, Inc. 2003. Minitab Statistical Software, Release 14 for Windows. State
College, PA.
66 Northeastern Naturalist Vol. 15, No. 1
Neufeld, H.S., and J.R. Renfro. 1993. Sensitivity of sycamore seedlings (Platanus
occidentalis) to ozone in Great Smoky Mountains National Park: Data from
1989. Natural Resources Report NPS/NRTR-93/131, Air Quality Division, US
National Park Service, Denver, CO. 39 pp.
Orendovici, T., J.M. Skelly, J.A. Ferdinand, J.E. Savage, M.-J. Sanz, and G.C. Smith.
2003. Response of native plants of northeastern United States and southern Spain
to ozone exposures: Determining exposure/response relationships. Environmental
Pollution 125:31–40.
Richards, B.L., J.T. Middleton, and W.B. Hewitt. 1958. Air pollution with relation to
agronomic crops. V. Oxidant stipple of grape. Agronomy Journal 50:559–561.
Skelly, J.M. 2000. Tropospheric ozone and its importance to forest and natural
plant communities of the northeastern United States. Northeastern Naturalist 7:
221–236.
Skelly, J.M., J.L. Innes, J.E, Savage, K.R. Snyder, D. VanderHeyden, J. Zhang, and
M.J. Sanz. 2000. Observation and confirmation of foliar ozone symptoms on native
plant species of Switzerland and southern Spain. Journal of Water, Air, and
Soil Pollution 116:227–234.
Umbach, D.M., and D.D. Davis. 1984. Severity and frequency of SO2-induced leaf
necrosis on seedlings of 57 tree species. Forest Science 30:587–596.
United States Congress (US Congress). 1977. The Clean Air Act as amended August
1977. P.L. 95–95. US Government Printing Office, Washington, DC.
United States Department of the Interior (US DOI). 2003. Ozone-sensitive plant species
on National Park Service and US Fish and Wildlife Service Lands: Results
of a June 24–25, 2003 Workshop, Baltimore, MD. Natural Resource Report NPS/
NRARD/NRR-2003/01.US DOI Publications, Denver, CO. 21 pp.
United States Environmental Protection Agency (US EPA). 1996. Air quality criteria
for ozone and related photochemical oxidants. Volume 1 of 3 reports EPA/600/P-
93/004aF, Research Triangle Park, NC.
VanderHeyden, D., J.M. Skelly, J. Innes, C. Hug, J. Zhang, W. Landolt, and P.
Bleuler. 2000. Ozone-exposure thresholds and foliar injury on forest plants in
Switzerland. Environmental Pollution 111:321–331.