Judging a Brook by its Cover:
The Relation Between Ecological Condition of a Stream
and Urban Land Cover in New England
James F. Coles, Thomas F. Cuffney, Gerard McMahon,
and Cornell J. Rosiu
Northeastern Naturalist, Volume 17, Issue 1 (2010): 29–48
Full-text pdf (Accessible only to subscribers.To subscribe click here.)

Access Journal Content
Open access browsing of table of contents and abstract pages. Full text pdfs available for download for subscribers.
Current Issue: Vol. 30 (3)
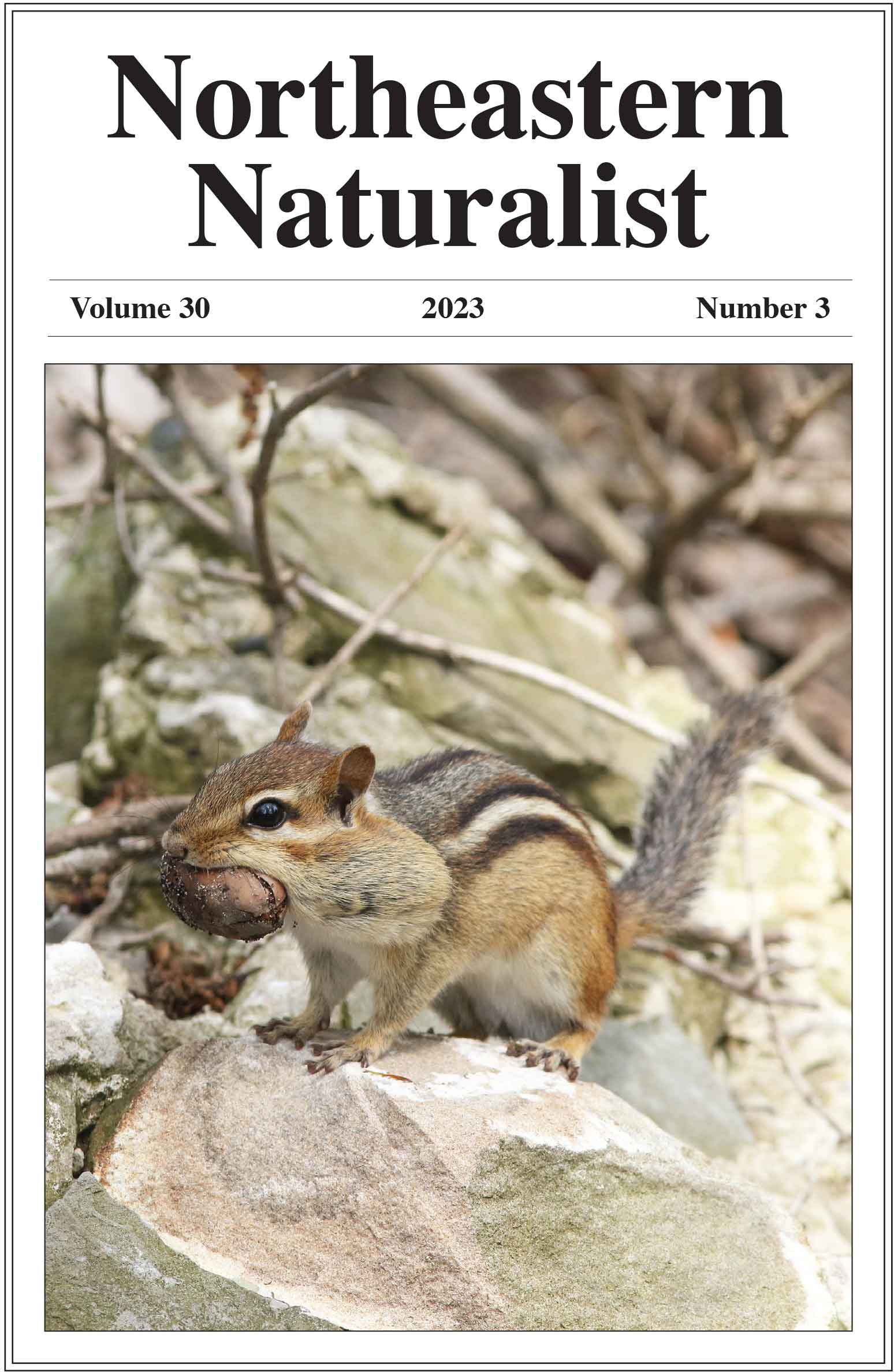
Check out NENA's latest Monograph:
Monograph 22
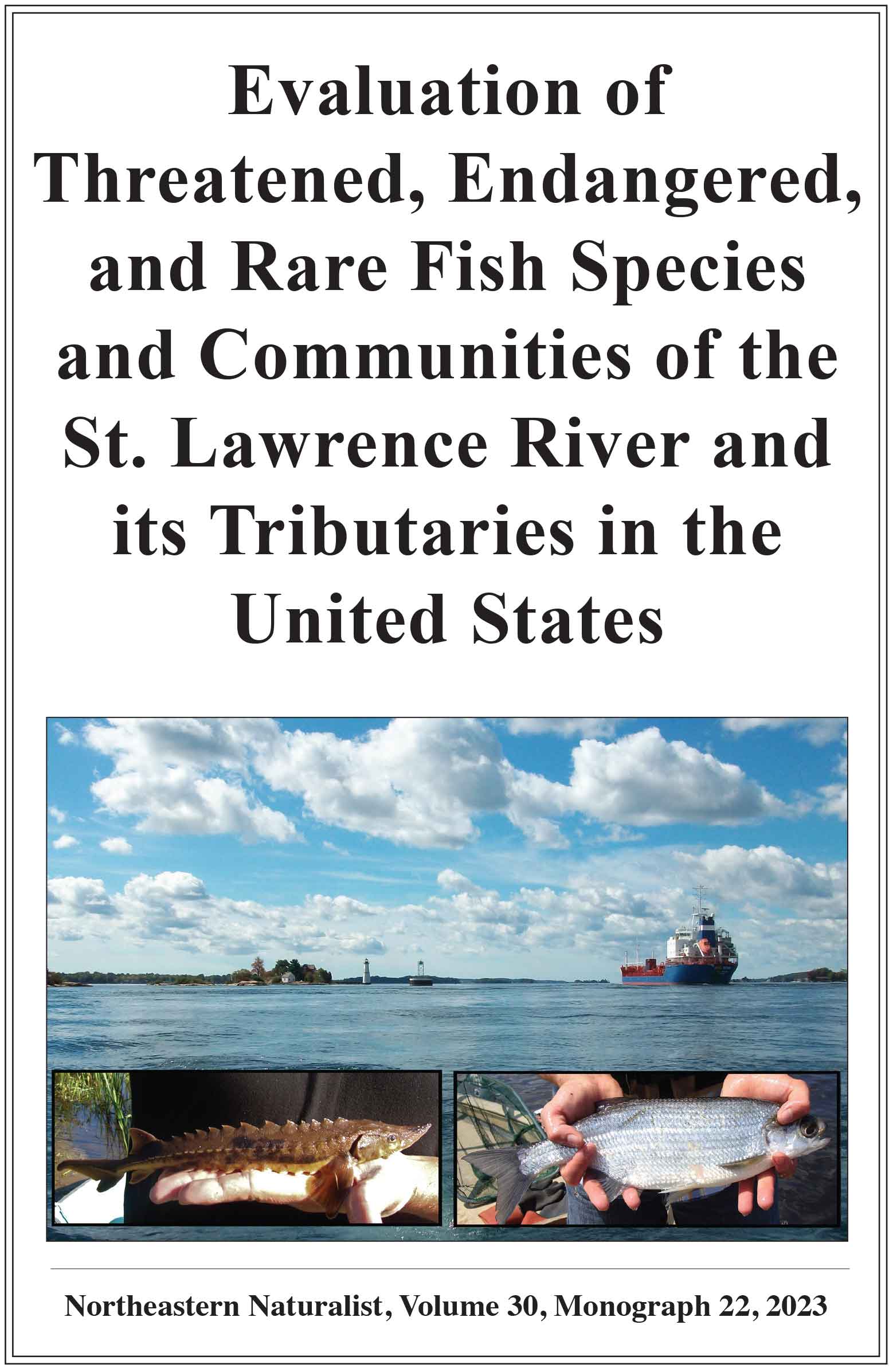
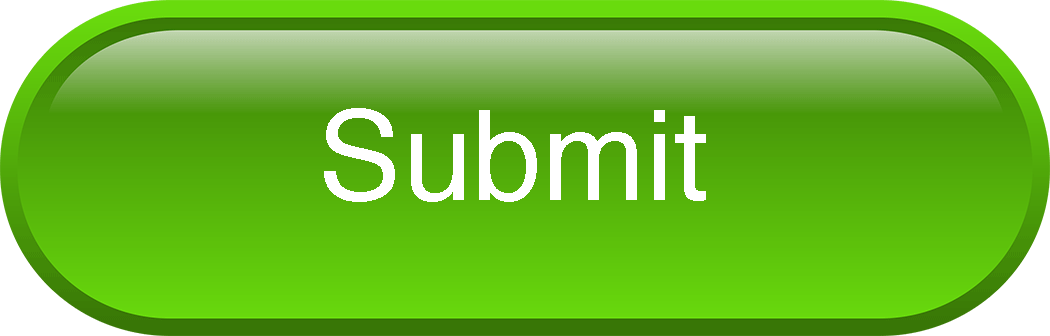
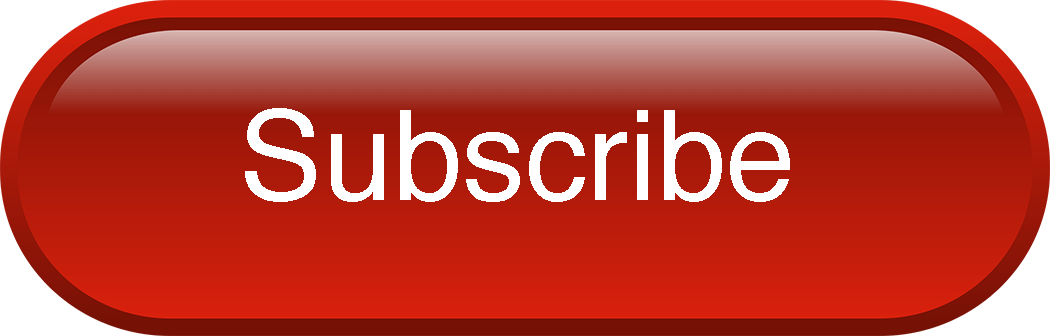
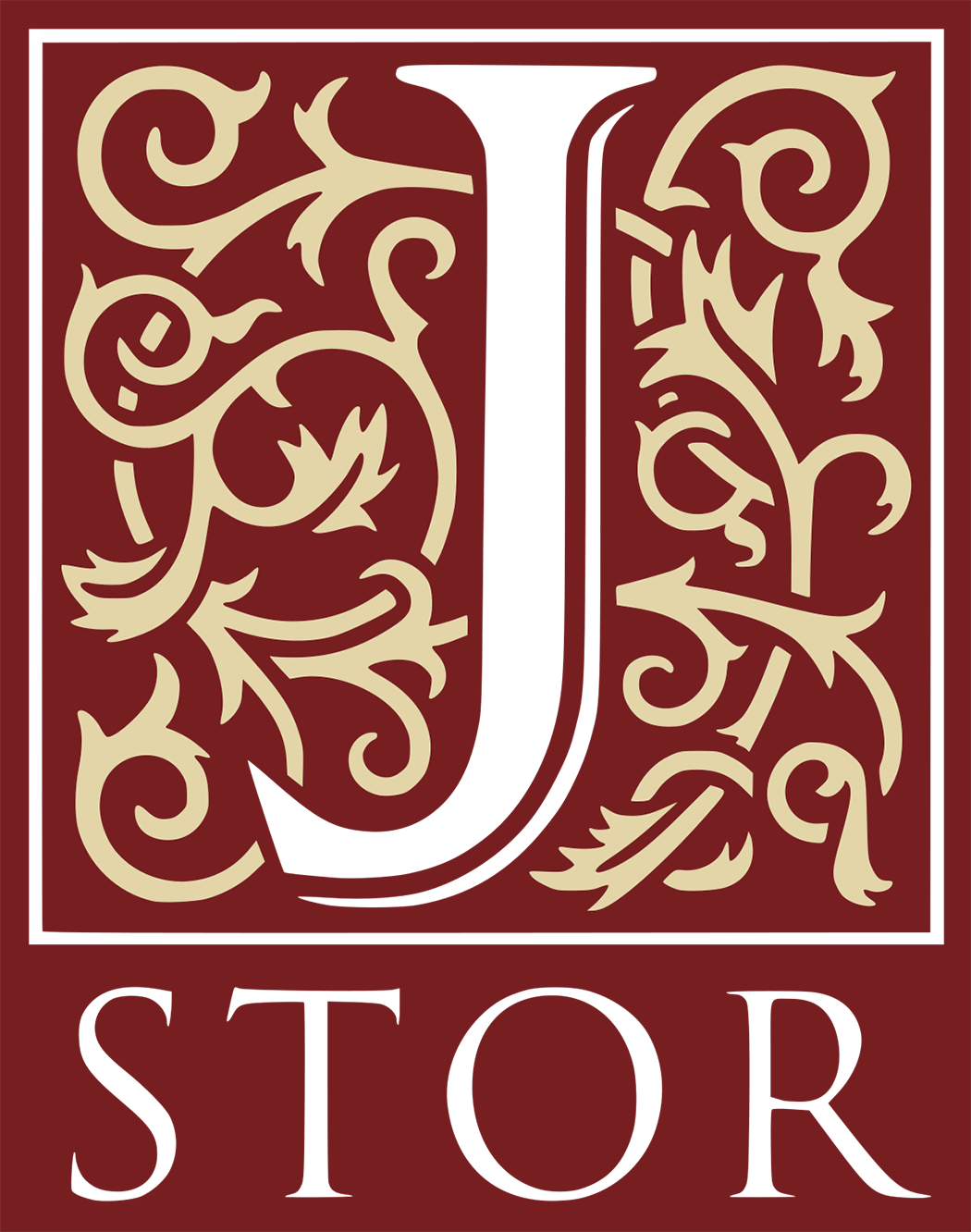
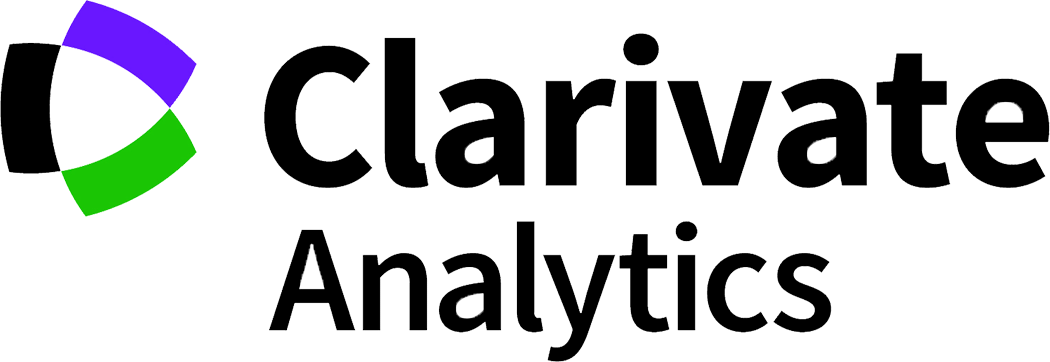
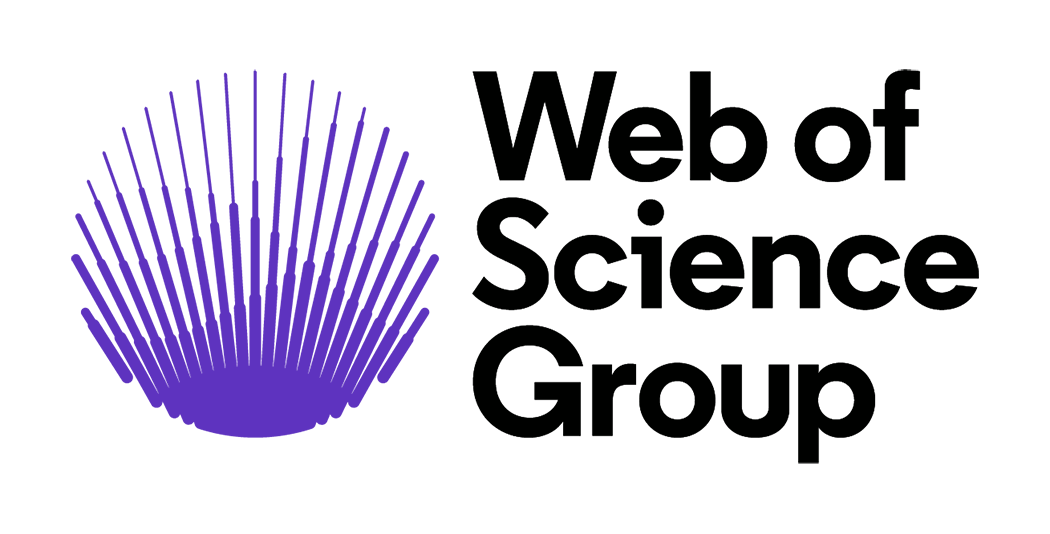
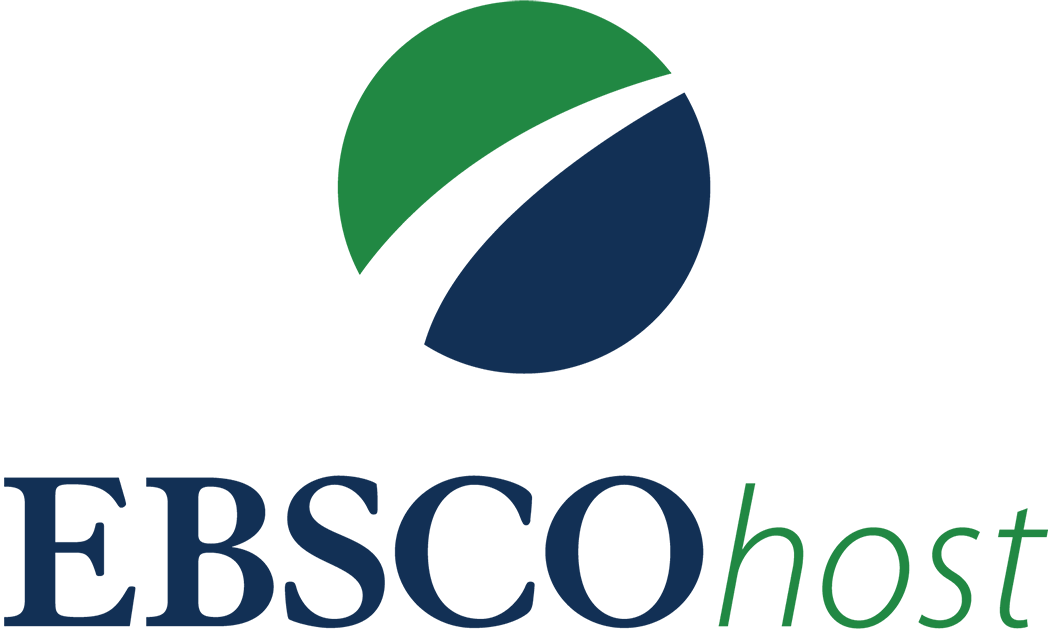
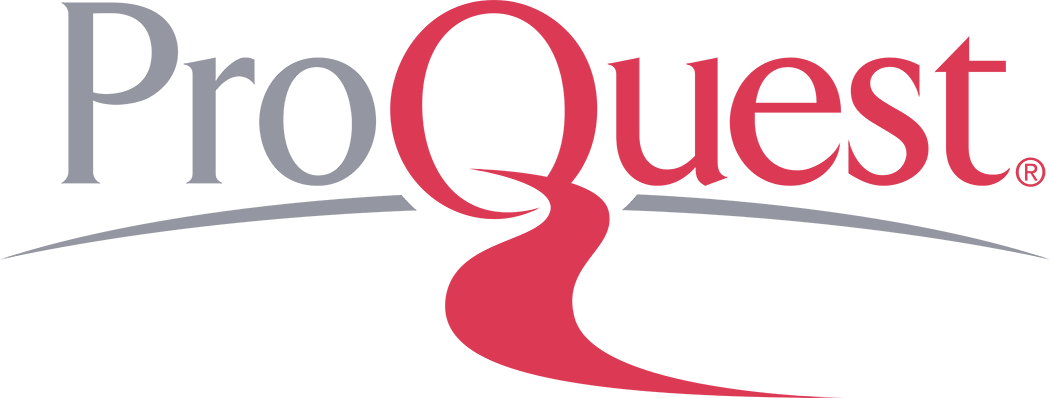
2010 NORTHEASTERN NATURALIST 17(1):29–48
Judging a Brook by its Cover:
The Relation Between Ecological Condition of a Stream
and Urban Land Cover in New England
James F. Coles1,*, Thomas F. Cuffney2, Gerard McMahon2,
and Cornell J. Rosiu3
Abstract - The US Geological Survey conducted an urban land-use study in the New
England Coastal Basins (NECB) area during 2001 to determine how urbanization relates
to changes in the ecological condition of streams. Thirty sites were selected that
differed in their level of watershed development (low to high). An urban intensity
value was calculated for each site from 24 landscape variables. Together, these 30
values reppresented a gradient of urban intensity. Among various biological, chemical,
and physical factors surveyed at each site, benthic invertebrate assemblages
were sampled from stream riffles and also from multiple habitats along the length of
the sampling reach. We use some of the NECB data to derive a four-variable urbanintensity
index (NECB-UII), where each variable represents a distinct component of
urbanization: increasing human presence, expanding infrastructure, landscape development,
and riparian vegetation loss. Using the NECB-UII as a characterization of
urbanization, we describe how landscape fragmentation occurs with urbanization and
how changes in the invertebrate assemblages, represented by metrics of ecological
condition, are related to urbanization. Metrics with a strong linear response included
EPT taxa richness, percentage richness of non-insect taxa, and pollution-tolerance
values. Additionally, we describe how these relations can help in estimating the
expected condition of a stream for its level of urbanization, thereby establishing a
baseline for evaluating possible affects from specific point-source stressors.
Introduction
The likelihood that urbanization of a watershed will cause some degree
of impairment to the receiving stream is generally acknowledged
by land planners and ecologists, but ways in which an aquatic system
responds and the level of urbanization at which responses occur are not
always understood (cf. Karr and Chu 1999, Paul and Meyer 2001, Pickett
et al. 2008, Schueler 1994, Walsh et al. 2005). In an urbanizing area, it
may not be apparent which particular features of landscape alteration are
the primary causes of ecological impairment to a stream. Alternatively,
when biological, physical, or chemical characteristics are used to assess
the condition of a stream, interpretations of the changes in these characteristics
are more precise when ways in which they relate to urbanization
are understood.
1US Geological Survey (USGS), 331 Commerce Way, Pembroke, NH 03275. 2USGS,
3916 Sunset Ridge Road, Raleigh, NC 27607. 3US Environmental Protection Agency
Region 1 New England, 1 Congress Street, Suite 1100 (HBS) Boston, MA 02114.
*Corresponding author - JColes@usgs.gov.
30 Northeastern Naturalist Vol. 17, No. 1
USGS urban land-use studies
From 2000 to 2004, the US Geological Survey (USGS) National Water-
Quality Assessment (NAWQA) program conducted urban land-use studies
to determine the effects of urbanization on stream ecosystems; these urban
studies focused on nine major metropolitan areas across the US, each associated
with a different physiographic region (Couch and Hamilton 2002,
Tate et al. 2005). The main objective of the studies was to gain a better
understanding of how urbanization affected stream ecosystems in a nationally
consistent manner by investigating the relation of urban intensity to
the biological, chemical, and physical condition of streams. The New England
Coastal Basins (NECB) region is a designated USGS study area that
includes most of Rhode Island and the eastern drainages of Connecticut,
Massachusetts, New Hampshire, and southern Maine. The NECB study area
includes metropolitan Boston, as well as some of the least-developed areas
in the eastern US (Ayotte and Robinson 1997). Consequently, the NECB
study area was one of the nine regions selected for an urban study. Details of
the NECB urban study are described in a USGS Professional Paper (Coles
et al. 2004), a summary of which is given below to provide the context for
the current paper. (Reports on the NECB study unit that are referenced in
this paper may be found online at http://nh.water.usgs.gov/projects/nawqa/
nawqaweb.htm.)
The NECB urban study was based on a network of sites consisting of 30
watersheds and their streams that were within 130 km from Boston, MA.
All sites were within US Environmental Protection Agency (USEPA) Ecoregion
59 (Northeastern Coastal Zone; Omernik 1987) and, additionally, were
within a select group of US Forest Service (USFS) ecological subsections
(Keys et al. 1995). The purpose of restricting the network of sites by these
ecologically defined areas was to help increase the certainty that differences
among sites could be attributed to urbanization rather than to natural variability.
The sites were further standardized by selecting third- to fifth-order
streams and delineating sampling reaches that were about 150 m long with
similar physical-habitat characteristics.
The level of urbanization was quantified for the 30 sites using a gradient
of urban intensity that collectively represented urbanizing watersheds by
substituting spatial for temporal variability. The gradient of urban intensity
was derived from 24 landscape variables that were related to different
urban-associated changes in the watersheds. These variables were selected
from datasets that characterized watersheds by three distinct categories:
basin land cover, human demography, and basin infrastructure. The basis of
selection was that a variable was correlated strongly to population density
(Spearman’s |rho| > 0.600), but correlated relatively weakly to watershed
size (|rho| < 0.400). The 24 variables were then equally weighted for use
in the gradient of urban intensity, which was then standardized over the 30
study sites from 0 to 100 to represent the lowest to highest levels of urban
intensity across the sites (McMahon and Cuffney 2000).
2010 J.F. Coles, T.F. Cuffney, G. McMahon, and C.J. Rosiu 31
The gradient of urban intensity derived for the NECB study was referenced
in Coles et al. (2004) as an a priori urban-intensity index (UII),
but it was used primarily to identify relations between urbanization and
biological, physical, and chemical characteristics of streams. It was not
designed to assign an urban-intensity value to watersheds within the
NECB area beyond the original study sites. However, because the a priori
UII had been shown to effectively characterize urbanization, it was relatively
simple to derive an index to measure the urban intensity for other
watersheds in the NECB area. Deriving the NECB urban intensity index
(NECB-UII) required two steps. First, the a priori urban gradient was
cumbersome with 24 variables, but this number could be reduced by removing
the less-relevant and redundant variables so that the NECB-UII
would use only a few variables and still function effectively. Second,
because the a priori urban gradient was scaled to function only with the
original 30 study sites, formulation of the NECB-UII would require that
the relation be expressed in a mathematical model for use in quantifying
urbanization at other sites.
Quantifying relations to urbanization
In a review of different approaches to rural land-use planning and
conservation, Theobald et al. (2005) described the need for developing
environmental indicators that are applicable to local planning efforts.
USEPA (2005) guidance for managing nonpoint source pollution in urbanizing
areas acknowledges that indicators for monitoring urban effects
vary by region and need to be specified. The guidance further defines
ecological measures needed for effective management of nonpoint pollution,
including characterizations of watershed conditions, and reliable
biological, physical, and chemical indicators for use in monitoring the effects
of urbanization.
Objectives of our paper are to use data from the NECB urban study to
(1) determine the general landscape changes that are related to urbanization
in the region, (2) derive an NECB-UII to quantify urbanization in the
watersheds, and (3) identify invertebrate-based metrics of ecological condition
that are related to urbanization. Additionally, a procedure is described
to estimate the expected ecological condition of a stream for a given level
of urban intensity, based on previously defined relations between urbanization
and invertebrate indicator metrics in the region. This approach would
have applications in ecological assessments of streams where it would be
difficult to assign impacts to a specific disturbance when compared to the
overall effects from watershed development (Rosiu and Coles 2005). For
example, when an ecological assessment is conducted at a site, invertebrateassemblage
metrics from the site could be compared to values expected for
the level of urbanization. Professional judgment could then be used to decide
if differences between actual and expected values infer a degraded stream
condition caused by a local disturbance (e.g., point source).
32 Northeastern Naturalist Vol. 17, No. 1
Methods
The 30 sites for the New England Coastal Basins (NECB) urban study
were selected during 1999 to represent a gradient of urban intensity from low
to high values, while ensuring that in-stream natural features were constant
among sampling reaches. Criteria for a sampling reach were that the stream
was free-flowing for at least 150 m with some riffles, had no sign of recent
human disturbance, and had well-defined banks with at least 50% mature
vegetation cover. With metropolitan Boston MA, as the primary urban
area, the sites collectively represented a gradient of urbanizing watersheds
(Fig. 1, Table 1).
Biological assemblages were sampled from August 1 to September 1,
2000. Semi-quantitative samples of aquatic invertebrates were collected
Figure 1. Site locations for the New England Coastal Basins (NECB) urban gradient
study and their association with the USEPA Level III Ecoregion and the USFS
Ecological Subsection.
2010 J.F. Coles, T.F. Cuffney, G. McMahon, and C.J. Rosiu 33
with a Slack sampler (0.25-m2 area, 425-micron net) from five riffle areas in
each sampling reach, which were composited and designated as the richest
targeted habitat (RTH) sample. A qualitative multihabitat (QMH) invertebrate
sample was also collected with a 212-micron mesh dipnet by sampling
various microhabitats along the 150-m sampling reach (Cuffney et al. 1993).
The RTH data were expressed as relative abundances. Additional taxa identified in the RTH sample but not found in the QMH sample were included in
Table 1. Location of the urban land-use sites, area of their watersheds, and values of the New
England Coastal Basins urban intensity index (NECB-UII). Site number corresponds to site
location on Fig. 1. WA = watershed area (km2), UII = NECB-UII value.
USGS Site Site
Station code # Site name and location WA UII
Maine
01072540 LIME 1 Little River near Lebanon 45.8 13.6
01072650 GREA 2 Greatworks River near North Berwick 60.2 23.4
New Hampshire
01072845 ISIN 3 Isinglass River at Batchelder Road near center 59.4 18.7
Strafford
01072904 BELL 4 Bellamy River at Bellamy Road near Dover 68.5 28.2
01073260 LAMP 5 Lamprey River at Cotton Road near Deerfield 83.1 14.6
01073458 NORT 6 North River at Rt 152 near Nottingham 74.9 17.5
010734833 LINH 7 Little River at Cartland Road at Lee 52.2 19.3
01089743 LSUN 8 Little Suncook River at Blackhall Road at Epsom 101.4 23.2
01090477 BLAB 9 Black Brook at Dunbarton Road near Manchester 53.7 16.9
01094005 BABO 10 Baboosic River at Bedford Road near Merrimack 73.0 24.1
010965852 BEAV 13 Beaver Brook at North Pelham 121.7 49.7
Massachusetts
01095220 STIL 11 Stillwater River near Sterling 78.7 21.0
01096544 STON 12 Stony Brook at School Street at Chelmsford 107.7 43.4
01096710 ASSA 14 Assabet River at Allen Street at Northborough 76.4 51.0
01096945 ELIZ 15 Elizabeth Brook off White Pond Road near Stow 48.5 28.4
01097270 FORT 16 Fort Pond Brook at River Road near South Acton 53.7 36.6
01097476 SUDB 17 Sudbury River at Concord Street at Ashland 89.6 40.3
01101500 IPSW 18 Ipswich River at South Middleton 115.3 63.7
01102345 SAUG 19 Saugus River at Saugus Ironworks at Saugus 60.4 96.7
01102500 ABER 20 Aberjona River (head of Mystic River) at 65.1 10.9
Winchester
011032058 CHAR 21 Charles River at Maple Street at North Bellingham 54.4 55.2
01105000 NEPO 22 Neponset River at Norwood 84.9 57.3
01105500 ENEP 23 East Branch Neponset River at Canton 72.9 66.6
01105581 MONA 24 Monatiquot River at River Street at Braintree 71.2 80.0
01106468 MATF 25 Matfield River at North Central Street at East 79.8 93.1
Bridgewater
01109000 WADE 26 Wading River (head of Threemile River) near Norton 113.4 39.3
01109595 MIDD 27 Middle River off Sutton Lane at Worcester 124.7 57.6
01110000 QUIN 28 Quinsigamond River at North Grafton 66.2 80.9
01112262 MILL 29 Mill River at Summer Street near Blackstone 73.7 31.5
Connecticut
01193340 BLAL 30 Blackledge River above Lyman Brook near North 49.2 24.8
Westchester
34 Northeastern Naturalist Vol. 17, No. 1
the QMH dataset. The final QMH dataset, therefore, expressed overall assemblage
richness along the sampling reach.
Addition data collected included fish and invertebrate-assemblage data,
habitat data, water-chemistry data, and stream-stage and water-temperature
data monitored continuously over about a year. These data are not discussed
in detail, but it is important to specify that there was relatively little
variance among sites in the habitat data. This result confirms that the studydesign
objective for heterogeneity among sampling reaches was successful,
and that ecological condition of the streams could be related to watershed
features with greater certainty. See Coles et al. (2004) for further details on
these data.
Selecting variables for the NECB-UII
The 24 landscape variables originally used in the NECB urban gradient
were evaluated by principal component analyses (PCA) to identify a smaller
set of variables that could define urbanization. By the nature of PCA, a variable
strongly associated with urbanization would have a strong loading value
(factor score on the first ordination axis) and a high fit statistic (correlation
along the first ordination axis). Additionally, variables in the reduced set
were categorized by specific but different aspects of urbanization: basin
land cover, human demography, and basin infrastructure. We considered that
including variables representing each of these categories was important for
the NECB-UII so that it would function as a proxy for many urban-related
changes. Therefore, the NECB-UII would be a more comprehensive representation
of urban intensity than any single variable and likely be more
responsive to various disturbances.
Variables selected for the NECB-UII required scaling coefficients before
they could be expressed collectively in a model used for calculating the
urban intensity of watersheds. The scaling coefficient for each variable was
its regression-line slope that was determined from the first-order (linear)
equation of the scaled values (0–100) over the raw values for the original
30 study sites. The scaling coefficients could then be used in the NECB-UII
to determine the urban intensity for any site by multiplying values of the
landscape variables by their scaling coefficients, then finding the average of
the products.
Identifying the effects of urbanization
The general change to the landscape from urbanization in the region was
evaluated using correlations of the NECB-UII to land-cover patch statistics.
Patch in this context represents a discrete area of homogeneous land cover
that is differentiated by discontinuities with the adjoining patches. Patch
density (the number of patches in a given area) analysis was done using
FRAGSTATS (McGarigal and Marks 1995) to determine the extent that the
landscape fragments as forested and developed land change in relation to
urban intensity.
2010 J.F. Coles, T.F. Cuffney, G. McMahon, and C.J. Rosiu 35
To evaluate the relations of invertebrate RTH (quantitative) and QMH
(qualitative) assemblages to urbanization, each dataset was first analyzed
with correspondence analyses (CA), and the resulting first-axis site scores
were correlated with the NECB-UII. Invertebrate metrics that were previously
found to respond to urban intensity (i.e., |rho| > 0.7; Coles et al. 2004)
were correlated with the NECB-UII. Included were metrics of abundance,
richness, diversity, dominance, functional groups, and tolerance, which are
commonly used in stream bioassessments (Barbour et al. 1999, Davis and
Simon 1995, Rosenberg and Resh 1993).
The USGS Invertebrate Data Analysis System (IDAS; Cuffney 2003)
was used to resolve taxa ambiguities in the invertebrate data and to calculate
the invertebrate metrics. Tolerance and functional-group metrics were
calculated in IDAS with attribute data from the USEPA Rapid Bioassessment
Protocol (Barbour et al. 1999). The multivariate ordinations were
done with CANOCO 4.0 (ter Braak and Smilauer 1998). The correlations,
regressions and scatterplots were done with SYSTAT 8 (SPSS 1998).
Spearman correlation coefficients > 0.7 (|rho|, absolute value) were considered
ecologically relevant.
Results
Deriving the urban intensity index
PCA results indicated how strongly each of the 24 landscape variables
contributed to the a priori urban gradient. First-axis loading values from
the PCA ranged from 0.535 to 0.977, and fit statistics ranged from 0.286 to
0.955. Of the variables, seven had loadings and fit statistics that were 0.9
or greater and these were deemed candidates for the UII (Table 2). Candidate
variables were judged as to how they were related to fundamentally
different landscape changes associated with urbanization. This process was
Table 2. Variables from the a priori urban gradient that were identified as candidate variables
for the New England Coastal Basins urban-intensity index (NECB-UII). Loading values and fit
statistics are results from PCA ordination axis-1, and indicate the relation of each variable to
the urban gradient. Variables with an “*” were used in the NECB-UII. NLCD, National Land
Cover Data.
Loading Fit
Variable Description value statistic
ROADDEN* Road density in watershed [road length (km)/watershed 0.975 0.950
area (km2)]
pBUF_2 Percentage of stream buffer (120 m outward from banks) 0.948 0.900
in developed land cover
pBUF_4* Percentage of stream buffer (120 m outward from banks) 0.950 0.903
in forest land cover
pNLCD_2* Percentage of watershed in NLCD “level 1” category: 0.970 0.940
developed
pNLCD_4 Percentage of watershed in NLCD “level 1” category: forest 0.977 0.955
pNLCD_21 Percentage of watershed in low-intensity residential 0.958 0.917
POP99DEN* Population density, 1999, people per hectare 0.953 0.909
36 Northeastern Naturalist Vol. 17, No. 1
useful for avoiding strong variable redundancies as well as defining variables
that would contribute to a comprehensive urban-intensity index. First
excluded was pNLCD_21 because it represents a highly specific land-cover
category that was inclusive with the general category pNLCD_2. Variables
for developed and forested land cover had a strong inverse relation (r2 =
0.954). Therefore, the land-cover associations of pBUF_2 to pBUF_4 and
of pNLCD_2 to pNLCD_4 were redundant, and consequently, only one
variable from each of these couplets would be considered a candidate for
the NECB-UII.
Each candidate variable was standardized over its range, and various
combinations of the variables were evaluated for the NECB-UII. Based on
the two-tiered procedure to identify variables by PCA that strongly related
to the a priori urban gradient, and that also represented specific aspects of
urbanization, variables selected were ROADDEN, pBUF_4, pNLCD_2,
and POP99DEN. The NECB-UII derived from these four variables was
strongly related to the a priori urban gradient (r2 = 0.966), and by advantage
of the four variables, would relate to the extents of infrastructure, loss
of stream buffer, watershed development, and human predominance on
the landscape. Additionally, when 21 invertebrate metrics were correlated
to the NECB-UII and to the a priori urban gradient, the coefficients were
overall slightly stronger with the NECB-UII (average |rho| = 0.860 and
0.849, respectively).
At this stage of development, the NECB-UII was still scaled only among
the study sites, as was the a priori urban gradient. However, by regressing
the absolute values against the scaled values of each variable, scaling coefficients were determined so that the NECB-UII could be expressed by the
following equation:
NECB-UII = ([(ROAD*12.5) + (BUFF%*1.74) + (DEV%*1.53) +
(POP *8.09)]*0.25),
where ROAD = road density (road length [km] / watershed area [km2]),
BUFF% = percentage stream buffer not in forest land cover (NLCD level 1),
DEV% = percentage watershed in developed land cover (NLCD level 1), and
POP = population density, people per hectare (US census data).
A consequence of this equation for the NECB-UII is that the urbanintensity
values across the 30 study sites change from 0 to 100 to a range
from 13.6 to 111. For example, the least urbanized site in the study (LIME;
Table 1) has a NECB-UII value of 13.6 instead of zero, because a zero value
would now represent a hypothetical site with no development.
Fragmentation of the landscape from urbanization
The predominant effect of urbanization on the landscape of the region
was the general loss of forest cover with encroaching development (Coles
et al. 2004). However, this shift was not a simple one-to-one parcel replacement
of forested land by developed land, as determined when the
changes in patch densities of the two land-use categories were compared.
2010 J.F. Coles, T.F. Cuffney, G. McMahon, and C.J. Rosiu 37
There was a linear increase in patch density (number of patches per area)
of forested land with increased urban intensity (rho = 0.941, Fig. 2a).
Concurrently, the average patch size decreased with urban intensity (rho
= -0.975), indicating that forests are lost from urbanization as they fragment
into smaller patches.
Patch density of developed land shows a different response pattern
(Fig. 2b). Over the urban-intensity gradient, the average patch size of developed
land increases consistently (rho = 0.952), but the number of the
patches increases only to a NECB-UII value of about 40 (rho = 0.872).
Above this value, patch density decreases with urban intensity (rho =
-0.859). These results suggest that urbanization of a forested watershed
begins with a few isolated small communities and that the number of
communities increases with urbanization only to a certain level. As urban
intensity continues to increase, communities appear to be coalescing into
fewer but larger towns and cities, which results in a decrease in the number
of developed-land patches. Forested land, however, continues to become
more fragmented with increasing urban intensity, possibly due in part to
concurrent increase in road density.
Invertebrate assemblage relations to urbanization
There were 294 invertebrate taxa identified in the samples collected from
the 30 sites. The CA ordinations of invertebrate data resulted in primaryaxis
eigenvalues of 0.350 for the RTH data and 0.324 for the QMH data,
indicating that both datasets had strong patterns of assemblage structure.
Furthermore, site scores from the RTH and QMH data ordinations were
strongly correlated to the NECB-UII (RTH, |rho| = 0.893; QMH, |rho| =
0.909) and they were relatively linear over the responses (Fig. 3A, B).
Several of the invertebrate metrics of structure and function that
are commonly used in biomonitoring programs were correlated to the
Figure 2. The relations between patch density of land cover and urban intensity
(NECB-UII). (A) Patches of forest vs. NECB-UII, rho = 0.941. (B) Patches of developed
land vs. NECB-UII, rho = 0.872 for the increasing response and rho = –0.859
for the decreasing response.
38 Northeastern Naturalist Vol. 17, No. 1
NECB-UII. Of approximately 100 metrics tested, those based on taxa
richness generally showed a stronger response than metrics based strictly
on abundance. These results indicated that qualitative information from
the QMH data was as relevant as the RTH abundance data in characterizing
relations of invertebrate assemblages to urbanization. This finding is
consistent with the results from the correlations of ordination site scores
to the NECB-UII (Fig. 3A, B) that indicated the RTH and QMH data were
strongly related to urbanization.
Total taxa richness was negatively correlated with the NECB-UII (rho =
-0.901) and with a linear response (Fig. 4A). Similarly, EPT taxa richness
was negatively correlated with the NECB-UII (rho = -0.902), even though
the number of EPT taxa accounted for less than half of the total taxa in every
sample (Fig 4B). Conversely, the percentage richness of non-insect taxa
increased linearly with increasing urban intensity (rho = 0.904; Fig. 4C). The
percentage richness of mollusks plus crustaceans (Mol + Crus), the dominant
non-insect taxa, also increased linearly with increasing urban intensity (rho
= 0.901; Fig. 4D).
Functional feeding-group metrics generally were not related as strongly
to urbanization as did metrics based on taxa structure. An exception was
richness of gatherer-collector taxa, which was negatively correlated with the
NECB-UII (rho = -0.800; Fig. 4E), and showed a “response-loss” threshold
at a NECB-UII value of about 50. A threshold such as this one indicates that
values of the metric are predictable only over a segment of the urban gradient,
which in this case was from low to moderate levels of intensity.
The average taxa tolerance, an average of pollution-tolerance values assigned
to taxa at a site, was linear and had a strong positive correlation with
the NECB-UII (rho = 0.892; Fig. 4F). This result indicated a gradual shift in
the invertebrate assemblages from sensitive to tolerant taxa with increasing
urban intensity.
Figure 3. The relation of invertebrate site scores to the NECB-UII. The site scores
were derived from Correspondence Analysis ordinations of the invertebrate data: (A)
RTH, quantitative sample from riffle habitats (|rho| = 0.893). (B) QMH, qualitative
sample from multiple habitats |rho| = 0.909).
2010 J.F. Coles, T.F. Cuffney, G. McMahon, and C.J. Rosiu 39
Although richness-based metrics generally had somewhat stronger
correlations to the NECB-UII than abundance-based metrics, hence the
usefulness of the QMH data, the quantitative RTH data were essential for
Figure 4. Relations of invertebrate taxa-richness metrics to the NECB-UII. These
metrics were based on the QMH (qualitative multihabitat) data. Spearman rho values
for each correlation: (A) total richness = -0.901, (B) EPT richness = -0.902, (C)
percentage richness of non-insects = 0.904, (D) percentage richness of mollusks plus
crustaceans = 0.901, (E) gatherer-collector richness = -0.800, (F) average taxa tolerance
= 0.892.
40 Northeastern Naturalist Vol. 17, No. 1
indices of diversity, evenness, and dominance. Shannon-Wiener diversity
index (SHANDIV) was negatively correlated with the NECB-UII (rho =
-0.867; Fig. 5A). In addition, the percentage abundance of the five most
dominant taxa (DOM5) was positively correlated with the NECB-UII (rho
= 0.870; Fig. 5B). A regression between SHANDIV and DOM5 showed
they were inversely related (r2 = 0.980), and suggests the response of
SHANDIV was influenced by the five dominant taxa. These responses
furthermore indicated that diversity and evenness of the taxa strongly decreased
with urbanization.
Discussion
The advantages of an urban-intensity index
Each of the four variables used in the NECB-UII represents a separate
aspect of an urbanizing watershed, and when combined in the NECB-UII,
functions as an effective proxy for various other changes associated with
urbanization. For example, population density has often been used as an indirect
measure of urban intensity, and accordingly, its use in the NECB-UII
is indicative of the general impact of human presence on the landscape. In
this regard, population density represents human activities in the watershed
that can affect stream condition. However, a concern in using a single variable
to characterize urbanization is that too much reliance is placed on one
indicator to respond to many changes that can cause stream impairment; a
multimetric index overcomes this uncertainty in part by relying on several
variables that function comprehensively to quantify urbanization.
Road density was used to characterize the infrastructure associated
with many of the human activities that contribute to urbanization, and has
been used as a surrogate for impervious surface (May et al. 1997). Of the
24 landscape variables used in the a priori urban gradient, road density
Figure 5. Relations of selected RTH invertebrate metrics to the NECB-UII. Spearman
rho values for the correlations: (A) Shannon-Wiener diversity = -0.867, (B) percentage
abundance of dominant 5 taxa = 0.870.
2010 J.F. Coles, T.F. Cuffney, G. McMahon, and C.J. Rosiu 41
generally correlated most strongly with site scores from the biological,
chemical, and physical datasets (Coles et al. 2004). Contaminants from
sources such as atmospheric deposition, vehicular traffic, and applications
of de-icing agents can accumulate on roadways and flush into streams,
thereby affecting water quality (Buckler and Granato 1999, Forman et al.
2002, Granato and Smith 1999). Biological assemblages can be directly
affected by these water-quality changes, but also by many of the physical
factors associated with roadways, such as increased sediment loads and
flow modifications to streams (Angermeier et al. 2004, Forman and Alexander
1998, Wood and Armitage 1997).
Percentage of watershed area in general developed land cover (NLCDLevel
I) was used to characterize the general change from a natural landscape
(typically forested for the region) to residential, commercial, and industrial
centers. Although land-cover classifications that represent more specific land
use (NLCD-Level II) were available, it was previously reported that correlations
between landscape change and biological, physical, and chemical
endpoints were usually stronger with the more comprehensive Level I classifications (Coles et al. 2004).
The percentage of stream buffer in forested cover was used to characterize
the loss of riparian forest that often is associated with urbanization. The
loss of forested cover along the riparian buffer was previously reported to
be associated with declining fish and invertebrate assemblages (Coles et al.
2004), and alterations in this zone probably affect stream condition more
directly than other land-cover variables. Although loss of forested cover
was closely related to increase in developed land cover for the watershed,
the loss of riparian forest helped to “weight” these disturbances by their
proximity to the stream. The importance of managing the natural riparian
corridor was recognized through legislation in Massachusetts as the Rivers
Protection Act of 1996 (Commonwealth of Massachusetts 1996), which
protects areas extending from the mean annual high-water line on banks of
perennial streams.
Landscape changes related to urbanization
The most notable landscape change related to urbanization in the region
was in how developed land increased as forested land was lost. It appeared
that urbanization resulted in a steady loss of forests by fragmenting the
natural landscape with patches of development. Fragmentation of forests
continued with urbanization, but beyond moderate levels of urban intensity
(NECB-UII > 40), the number of developed patches began decreasing as
they apparently coalesced into increasingly larger urban centers.
Impervious surface area (ISA) is closely associated with urbanization
and has been used to measure urban intensity in different regions (Schueler
1994). Values of ISA were not available at the time of our study, but establishing
the relation between the NECB-UII and ISA was considered important
so that our results could be compared to studies that use ISA as a surrogate
for urbanization. Subsequent to our study, estimated values of impervious42
Northeastern Naturalist Vol. 17, No. 1
surface data were derived by NOAA from mid-1990s satellite night-light
observations, 1992 land-cover data, and 2000 Census road-density data
(Elvidge et al. 2004, NOAA 2006). The NOAA-based ISA values for our
sites were used in a regression with the NECB-UII, and the resulting relation
was determined: percentage ISA = 0.382 * NECB-UII -4.27 (r2 = 0.982, P <
0.001)). Although a strong linear relation was confirmed, the regression line
intersects the NECB-UII axis at 11.2 (x-intercept value), which may indicate
that the relation is nonlinear over low values (Fig. 6).
Stream condition indicators of urbanization
The overall results of this study showed that urbanization was associated
with declining ecological conditions, which is consistent with results reported
in other studies (Kennen 1999, May et al. 1997, Morley and Karr 2002,
Walsh et al. 2005). Among the responses in the invertebrate-assemblage
data, changes in taxa richness appeared more important than abundance.
This finding was most strongly supported by the qualitative QMH data,
where the CA site scores and the metrics such as EPT richness and pollution
tolerance of taxa were strongly correlated to the NECB-UII.
In relating the effects of urbanization to invertebrate assemblages, a presumed
ecological “coupling” exists across spatial scales such that watershed
development causes changes to water quality that will ultimately affect assemblages
at the stream-reach level (Picket et al. 2008). A gradient of water
quality was described in Coles et al. (2004) that was PCA-derived from
water-chemistry data collected at the 30 study sites. Among water-quality
constituents that increased with urbanization were specific conductance,
total Kjeldahl nitrogen (TKN), total phosphorus, and total pesticides. Subsequent
analyses showed that the NECB-UII was strongly correlated to this
water-quality gradient (rho = -0.927), indicating that water quality declined
with urbanization. The water-quality gradient was also strongly correlated
to invertebrate metrics such as EPT richness (rho = 0.878), so it is likely
that water quality was an intermediate factor between urbanization and the
invertebrate response.
Figure 6. Relation between derived
values of impervious surface area (ISA)
and the NECB-UII.
2010 J.F. Coles, T.F. Cuffney, G. McMahon, and C.J. Rosiu 43
Interpreting responses to urbanization
Changes in the invertebrate assemblages generally began as soon as
conditions departed from background (minimal urban), which suggests that
they did not show a resistance to initial impairment as has been observed
in other studies (Cuffney et al. 2000). This result may indicate that the biological
communities are highly sensitive to watershed change, or that urban
intensity at “background conditions” was already at a level where impairment
would occur. The lowest urban-intensity value in the study (NECB-UII
= 13.6) indicates some degree of ecological disturbance may have occurred
in even the least urbanized watersheds in the region. Additionally, if the
relation between ISA and NECB-UII holds true (described above), a threshold
of disturbance at some critical ISA value was not apparent in how the
invertebrate assemblages responded over very low ISA values. This result
also indicates that the ISA level at which ecological disturbances first occur
is less than the sometimes-cited values of 10–15 percent (cf. Arnold and Gibbons
1996, Booth and Jackson 1997, Booth et al. 2004, Klein 1979, May et
al.1997, Schueler 1994).
Although variables did not show an initial resistance to urbanization,
we observed examples where the response was linear from low to moderate
urban intensities, but which then declined or even reached an exhaustion
phase at higher urban intensities. An example of this response-loss type of
threshold was observed in gatherer-collector richness for the invertebrates
(Fig. 4e), and has been described for taxa richness in benthic diatoms and
abundance of insectivorous fish (Coles et al. 2004). Response-loss thresholds
occurred at about the same level of urban intensity (NECB-UII around 50),
which was just above the level where the number of developed land patches
changed from increasing to decreasing with urbanization (Fig. 2b). It was
not discernable from the study, however, whether these thresholds indicated
a sensitivity of biological assemblages to effects from specific landscape
changes at that urban intensity, such as coalescing of developed patches into
urban centers.
Because thresholds are points along a response gradient where the underlying
relation changes abruptly, it is important that they be recognized when
assessing or monitoring aquatic resources. For example, if urban intensity
were to increase from mid- to high levels, an assessment of changing stream
condition would be different if based on the gatherer-collector richness
(Fig. 4E) compared to the average taxa tolerance (Fig. 4F). Although the use
of metrics that show a threshold response might be considered less effective
for assessing ecological condition, this information is still very useful for
identifying the level of a disturbance below which certain taxa can survive.
This situation could occur where the protection of particular species is
important, and the threshold value would indicate the urban intensity where
extirpation would be expected.
Compared to variables showing a threshold response, variables that
indicate long linear responses are often favored when assessing the
44 Northeastern Naturalist Vol. 17, No. 1
ecological condition of streams because they are more predictable over
a disturbance gradient. Examples from our study (all based on qualitative
data) were EPT taxa richness, percentage richness of non-insect taxa,
and the average taxa tolerance (Table 3). Each of these was derived from
a combination of at least three single-parameter variables (i.e., multiple
invertebrate taxa), which emphasizes that multimetric indices often more
effectively indicate a continuous response over a disturbance gradient than
a single variable can indicate.
Predicting effects from urbanization
EPA Region 1 (New England) recently initiated a project through the
Regional Applied Research Effort (RARE) of the Office of Science Policy
(USEPA 2007a) to evaluate the relations between land-cover variables
and biological responses, using data from the NECB urban gradient study
and the New England Wadeable Streams Survey (USEPA 2006, 2007b). In
addition, the biological responses investigated for this RARE project are
to be evaluated in the context of the Biological Condition Gradient (BCG),
a descriptive model in which a suite of ecological attributes are predicted
to change in response to increasing levels of stressors (Davies and Jackson
2006). The results are expected to determine the extent that ecological responses,
such as those we have described, are applicable to sites across the
region (N.E. Detenbeck, US EPA, June 2008 pers. comm.).
Results from our study may help in understanding the underlying relations
between urbanization and ecological response variables. It is anticipated
that our findings will be useful in assigning values of the expected
ecological condition for streams, given the level of urbanization in their
watersheds. For example, for a degraded stream segment undergoing restoration,
the expected condition after recovery could depend in large part
on urban intensity above the site. Using the relations between invertebrate
metrics and the NECB-UII (Fig. 4, Table 3), if the urban intensity of the
watershed was NECB-UII = 20, it would be reasonable to expect an EPT
richness of around 20 to 30 after restoration. Achieving EPT richness in
this range would be unrealistic, however, if the urban intensity was around
NECB-UII = 80. In this case, it is more likely that EPT values below 10
would be achieved. Therefore, the ability to predict the condition at a site
by accounting for “background” effects of urbanization is expected to
Table 3. Regression statistics for selected invertebrate metrics that have a strong linear relation
to urbanization as represented by the NECB-UII. Metrics are based on qualitative multihabitat
(QMH data), which characterizes assemblage richness (taxa presence/absence).
Invertebrate Probability Regression Y Figure
metric R2 value slope intercept in text
EPT richness 0.743 <0.001 -0.294 30.70 4b
Non-insect % richness 0.837 <0.001 0.342 4.45 4c
Average taxa tolerance 0.824 <0.001 0.022 3.92 4f
2010 J.F. Coles, T.F. Cuffney, G. McMahon, and C.J. Rosiu 45
improve the precision of stream assessments, especially when evaluating
effects from specific stressors.
Acknowledgments
The authors thank the many USGS colleagues who participated in the fieldwork
and the extensive data-collection efforts of the original study; special thanks go to
Karen Beaulieu of the USGS Connecticut Water Science Center, who coordinated
most of the fieldwork, kept track of the data, and assisted in the analysis. We greatly
appreciate the efforts of Mary C. Freeman (USGS), Cathy M. Tate (USGS), and Gary
Kleppel (University of Albany, SUNY) for technical reviews of the manuscript. Support
from EPA Region 1 was provided to J.F. Coles to serve as technical advisor on
the RARE project described above and to produce a draft of this paper. We recognize
Naomi Detenbeck (EPA/ORD Laboratory, Narragansett, RI) for her efforts in using
data from the NECB urban study in conjunction with regional EPA data to investigate
possible causes of impairments to aquatic systems in the region.
Literature Cited
Angermeier, P.A., A.P Wheeler, and A.E. Rosenberger. 2004. A conceptual framework
for assessing impacts of roads on aquatic biota. Fisheries 29:19–29.
Arnold, C.L., and C.J. Gibbons. 1996. Impervious surface coverage: The emergence
of a key environmental indicator. American Planners Association Journal
62:243–258.
Ayotte, J.D., and K.W. Robinson. 1997. National water-quality assessment program:
New England Coastal Basins. US Geological Survey Fact Sheet FS-060-97.
Available online at http://pubs.water.usgs.gov/fs060-97. Accessed January 2010.
4 pp.
Barbour, M.T., J. Gerritsen, B. D. Snyder, and J.B. Stribling. 1999. Rapid bioassessment
protocols for use in streams and wadeable rivers: Periphyton, benthic
macroinvertebrates, and fish (2d Edition). US Environmental Protection Agency,
Office of Water, EPA 841-B-99-002, Washington, DC.
Booth, D.B., and C.R. Jackson. 1997. Urbanization of aquatic systems: Degradation
thresholds, stormwater detection, and the limits of mitigation. Journal of the
American Water Resources Association 33(5):1077–1090.
Booth, D.B, J.R. Karr, S. Schauman, C.P. Konrad, Christopher, S.A. Morley, M.G.
Larson, and S.J. Burges. 2004. Reviving urban streams: Land use, hydrology,
biology, and human behavior. Journal of the American Water Resources Association
40:1351–1363.
Buckler, D.R., and G.E. Granato. 1999. Assessing biological effects from highway
runoff constituents. US Geological Survey Open-File Report 99-240, Northborough,
MA.
Coles, J.F., T.F. Cuffney, G. McMahon, and K.M. Beaulieu. 2004. The effects of
urbanization on the biological, physical, and chemical characteristics of coastal
New England streams. US Geological Survey Professional Paper 1695, Northborough,
MA.
Commonwealth of Massachusetts. 1996. 310 Code of Massachusetts Regulations
(CMR) 10.00, chapter 258.
46 Northeastern Naturalist Vol. 17, No. 1
Couch, C., and P. Hamilton. 2002. Effects of urbanization on stream ecosystems. US
Geological Survey Fact Sheet 042-02, Reston, VA.
Cuffney, T.F. 2003. User's manual for the National Water-Quality Assessment program
invertebrate data analysis system (IDAS) software—Version 3. US Geological
Survey Open-File Report 03-172, Raleigh, NC.
Cuffney, T.F., M.E. Gurtz, and M.R. Meador. 1993. Methods for collecting benthic
invertebrate samples as part of the National Water Quality Assessment Program.
US. Geological Survey Open File Report 93-406, Raleigh, NC.
Cuffney, T.F., M.R. Meador, S.D. Porter, and M.E. Gurtz. 2000. Responses of physical,
chemical, and biological indicators of water quality to a gradient of agricultural
land use in the Yakima River Basin, Washington. Environmental Monitoring
and Assessment 64:259–270.
Davies, S.P., and S.K. Jackson. 2006. The biological condition gradient: A descriptive
model for interpreting change in aquatic ecosystems. Ecological Applications
16(4):1251–1266.
Davis, W.S., and T.P. Simon. 1995. Biological Assessment and Criteria: Tools
for Water Resource Planning and Decision Making. Lewis Publishers, Boca
Raton, fl.
Elvidge, C.D., C. Milesi, J.B. Dietz, B.T. Tuttle, P.C. Sutton, R. Nemani, and J.E.
Vogelmann. 2004. US constructed area approaches the size of ohio. Eos, Transactions
of the American Geophysical Union 85, 24, 233.
Forman, R.T.T., and L.E. Alexander. 1998. Roads and their major ecological effects.
Annual Review of Ecology and Systematics 29:207–231.
Forman, R.T.T., D. Sperling, J.A. Bissonette, A.P. Clevenger, C.D. Cutshall, V.H.
Dale, L. Fahrig, R. France, C.R. Goldman, K. Heanue, J.A. Jones, F.J. Swanson,
T. Turrentine, and T.C. Winter. 2002. Road Ecology: Science and Solutions. Island
Press, Washington, DC.
Granato, G.E., and K.P. Smith. 1999. Estimating concentrations of road-salt constituents
in highway-runoff from measurements of specific conductance. US
Geological Survey Water-Resources Investigations Report 99-4077, Northborough,
MA.
Karr, J.R., and E.W. Chu. 1999. Restoring Life in Running Waters: Better Biological
Monitoring. Island Press, Washington, DC.
Kennen, J.G. 1999. Relation of macroinvertebrate community impairment to catchment
characteristics in New Jersey streams. Journal of the American Water Resources
Association 36(4):939–955.
Keys, J.E., Jr., C.A. Carpenter, S.L. Hooks, F.G. Koenig, W.H. McNab, W.E. Russell,
and M.L. Smith. 1995. Ecological units of the eastern United States—First
approximation: Atlanta, GA (map [scale 1:3,500,000] and booklet of tables). US
Department of Agriculture, Forest Service. Available online at http://www.fs.fed.
us/rm/ecoregions/. Accessed January 2010. 80 pp.
Klein, R.D. 1979. Urbanization and stream quality impairment. Water Resources
Bulletin 15:948–963.
May, C.W., R. Horner, J.R. Karr, B. Mar, and E. Welch. 1997. Effects of urbanization
on small streams in the Puget Sound Lowland Ecoregion. Watershed Protection
Techniques 2(4):483–494.
2010 J.F. Coles, T.F. Cuffney, G. McMahon, and C.J. Rosiu 47
McGarigal, K., and B.J. Marks. 1995. FRAGSTATS: Spatial pattern analysis
program for quantifying Landscape Structure. US Department of Agriculture,
Forest Service, Pacific Northwest Research Station. General Technical Report
PNW-GTR-351. Available online at http://www.treesearch.fs.fed.us/pubs/3064.
Accessed January 2010.
McMahon, G., and T.F. Cuffney. 2000. Quantifying urban intensity in drainage
basins for assessing stream ecological conditions. Journal of American Water
Resources Association 36(6):1247–1261.
Morley, S.A., and J.R. Karr. 2002. Assessing and restoring the health of urban
streams in the Puget Sound Basin. Conservation Biology 16(6):1448–1509.
National Oceanic and Atmospheric Administration (NOAA). 2006. Impervious surface
area of the United States. Available online at http://www.csc.noaa.gov/crs/
lca/ccap.html. Accessed July 2006.
Omernik, J.M. 1987. Ecoregions of the conterminous United States. Annals of the
Association of American Geographers 77(1):118–125.
Paul, M.J., and J.L. Meyer. 2001. Streams in the urban landscape. Annual Review of
Ecology and Systematics 32:333–365.
Pickett, S.T.A., M.L. Cadenasso, J.M. Grove, P.M. Groffman, L.E. Band, C.G.
Boone, W.R. Burch, C.S.B. Grimmond, J. Hom, J.C. Jenkins, N.L. Law, C.H.
Nilon, R.V. Pouyat, K. Szlavecz, P.S. Warren, and M.A. Wilson. 2008. Beyond
urban legends: An emerging framework of urban ecology, as illustrated by the
Baltimore Ecosystem Study. Bioscience 58(2):139–150.
Rosenberg, D.M., and V.H. Resh. 1993. Freshwater Biomonitoring and Benthic Macroinvertebrates.
Chapman and Hall, Inc., Routledge, New York, NY.
Rosiu, C.J, and J.F. Coles. 2005. Standardizing sediment risk characterization on
the basis of urban intensity of the watershed. Poster presented at EPA Science
Forum, May 18, 2005. Collaborative Science for Environmental Solutions,
Washington, DC.
Schueler, T. 1994. The importance of imperviousness. Watershed Protection
1(3):100–111.
SPSS. 1998. SYSTAT 8.0 Statistics. SPSS Inc., Chicago, IL.
Tate, C.M., T.F. Cuffney, G. McMahon, E.M.P. Giddings, J.F. Coles, and H. Zappia.
2005. Use of an urban-intensity index to assess urban effects on streams in three
contrasting environmental settings. Pp. 291–315, In L.R. Brown, R.M. Hughes,
R. Gray, and M.R. Meador (Eds.). Effects of Urbanization on Stream Ecosystems.
American Fisheries Society, Symposium 47, Bethesda, MD.
ter Braak, C.J.F. and P. Smilauer. 1998. CANOCO manual and user’s guide to
CANOCO for windows. Software for CANOCO community ordination (Version
4). Microcomputer Power, Ithaca, NY.
Theobald, D.M., Thomas Spies, J.D. Kline, Bruce Maxwell, N.T Hobbs, and V.H.
Dale. 2005. Ecological support for rural land-use planning. Ecological Applications
15(6):1906–1914.
US Environmental Protection Agency (USEPA). 2005. National management measures
to control nonpoint source pollution from urban areas. USEPA, Office of
Wetlands, Oceans, and Watersheds, Washington, DC. EPA-841-B-05-004.
USEPA. 2006. Wadeable Streams Assessment: A Collaborative Survey of the Nation’s
Streams. USEPA, Office of Wetlands, Oceans, and Watersheds, Washington,
DC. EPA-841-B-06-002.
48 Northeastern Naturalist Vol. 17, No. 1
USEPA. 2007a. Regional Applied Research Effort (RARE) Program homepage. Available
online at http://www.epa.gov/osp/regions/rare.htm. Accessed 7 June 2007.
USEPA. 2007b. The New England Wadeable Stream Survey (NEWS): Development
of common assessments in the framework of the biological condition gradient.
Available online at http://www.epa.gov/ne/lab/pdfs/NEWSfinalReport_August2007.
pdf. Accessed October 2007.
Walsh, C.J, A.H. Roy, J.W. Feminella, P.D. Cottingham, P.M. Groffman, and R.P.
Morgan. 2005. The urban-stream syndrome: Current knowledge and the search
for a cure. Journal of the North American Benthological Society 24:706–723
Wood, P.J., and P.D. Armitage. 1997. Biological effects of fine sediment in the lotic
environment. Environmental Management 21:203–217.