Mycorrhizal Colonization of Hypericum perforatum L.
(Hypericaceae) from Serpentine and Granite Outcrops on
the Deer Isles, Maine
Naveed Davoodian, Jason Bosworth, and Nishanta Rajakaruna
Northeastern Naturalist, Volume 19, Issue 3 (2012): 517–526
Full-text pdf (Accessible only to subscribers.To subscribe click here.)
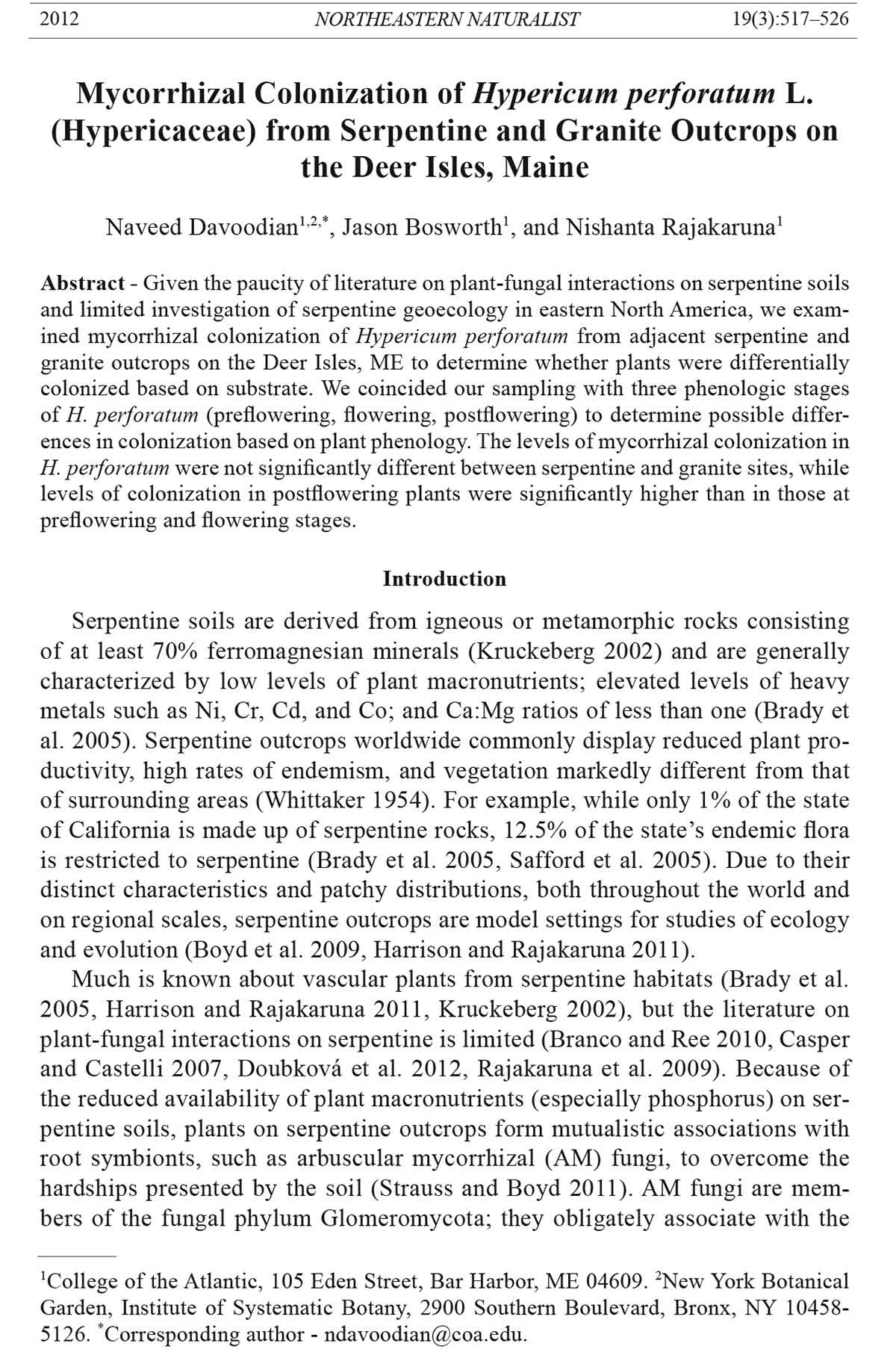
Access Journal Content
Open access browsing of table of contents and abstract pages. Full text pdfs available for download for subscribers.
Current Issue: Vol. 30 (3)
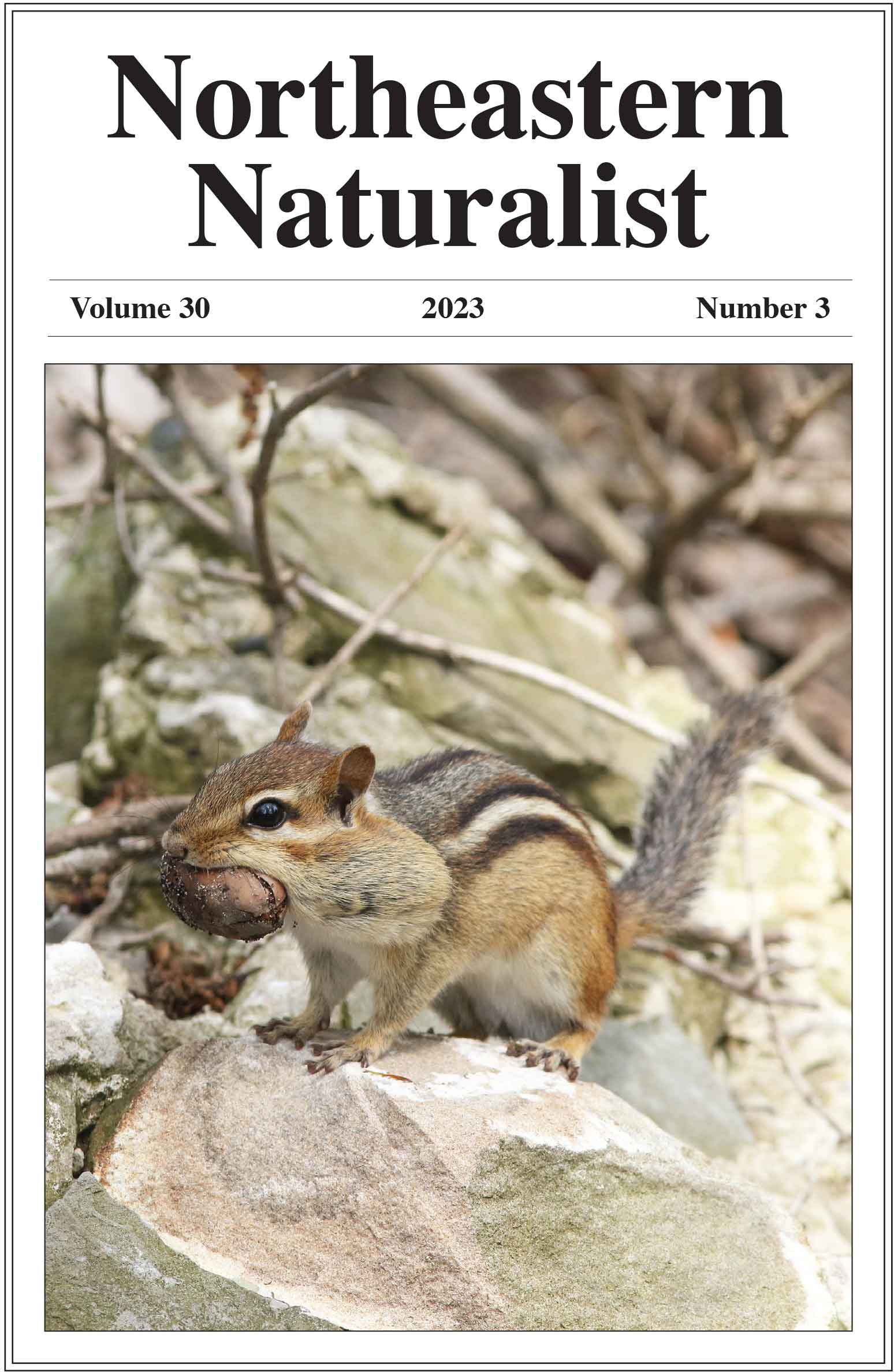
Check out NENA's latest Monograph:
Monograph 22
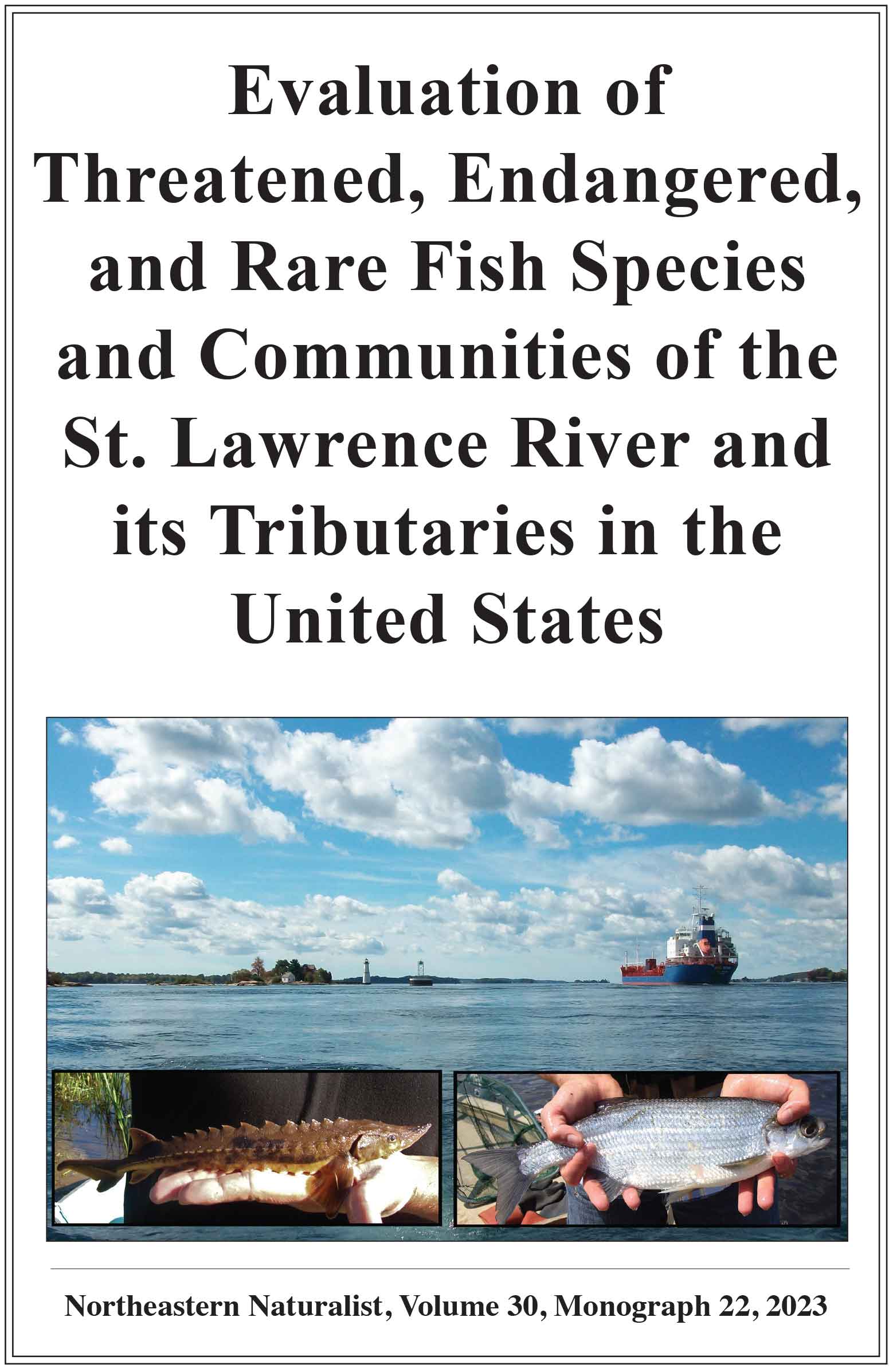
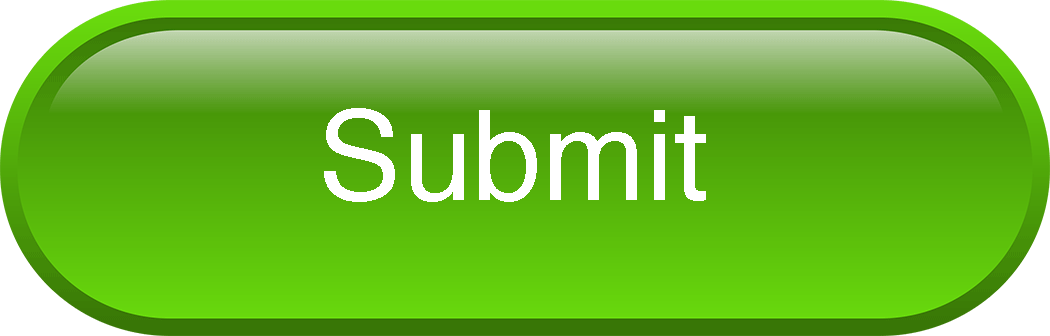
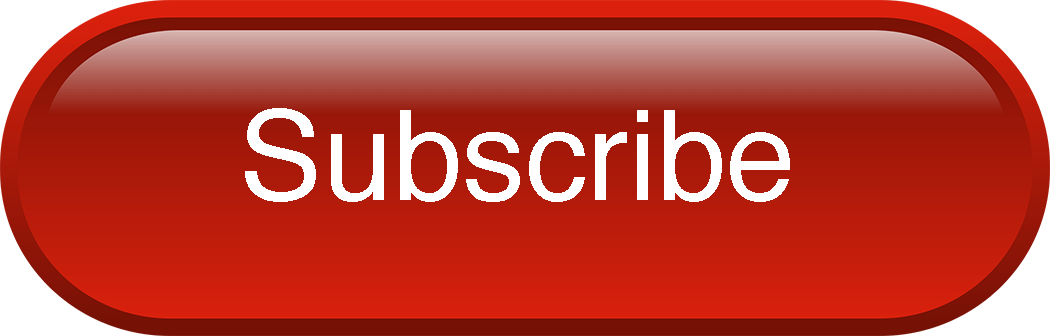
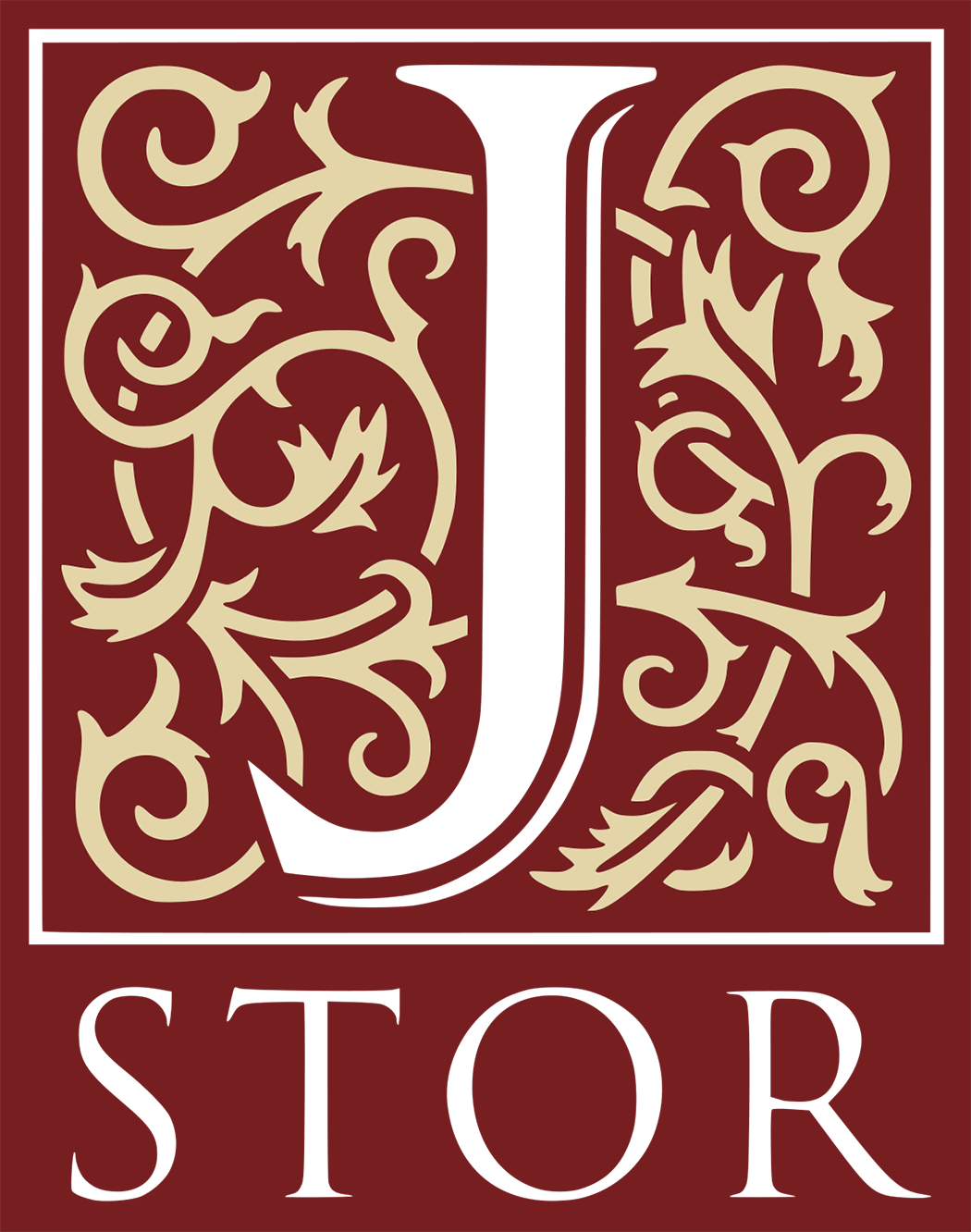
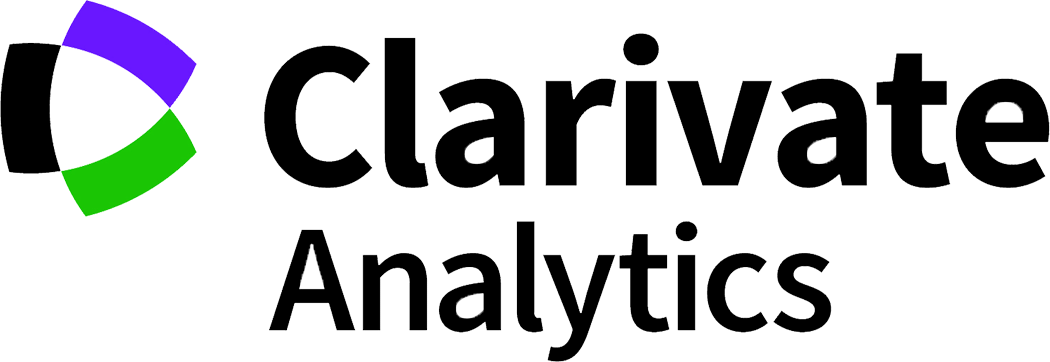
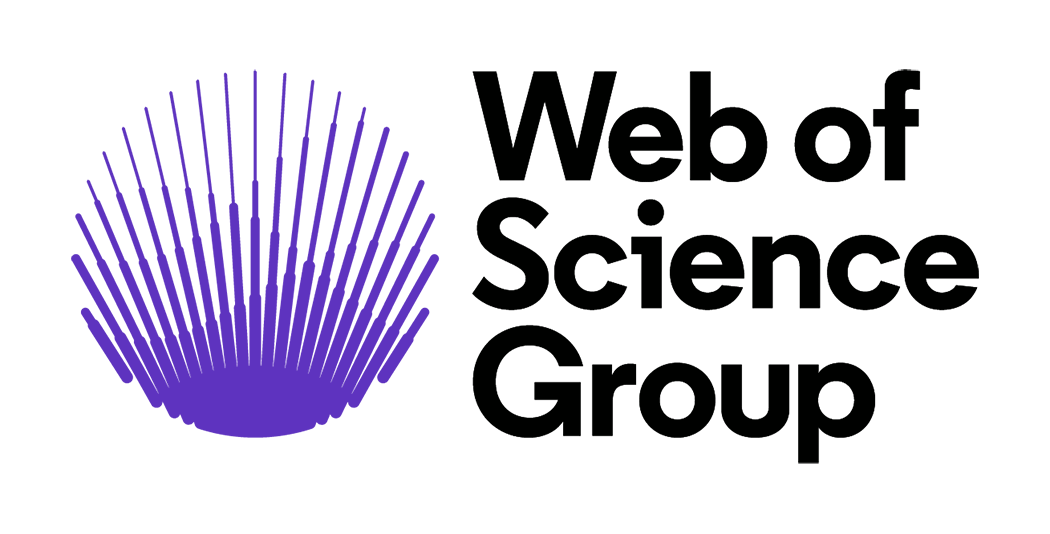
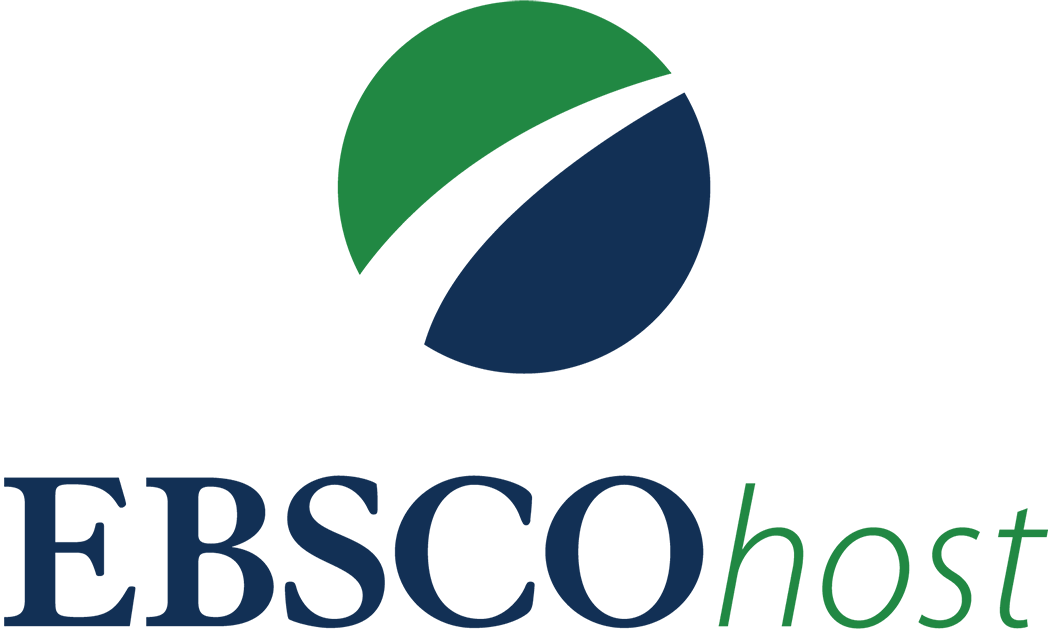
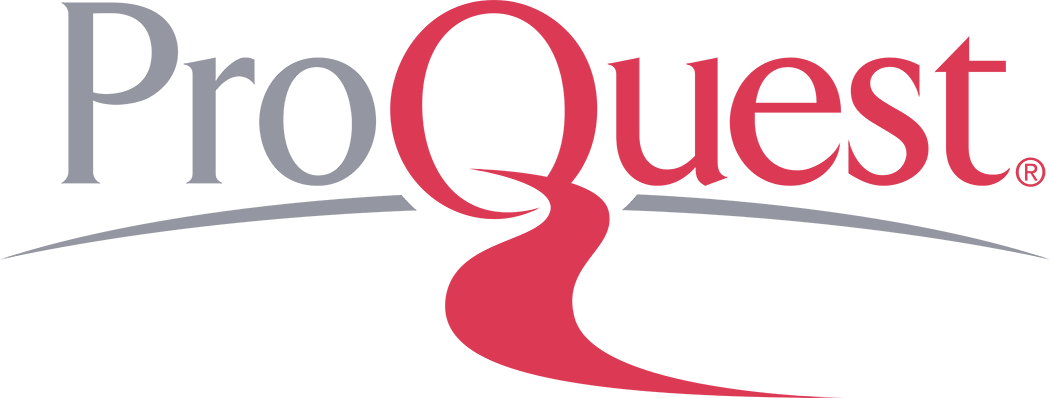
2012 NORTHEASTERN NATURALIST 19(3):517–526
Mycorrhizal Colonization of Hypericum perforatum L.
(Hypericaceae) from Serpentine and Granite Outcrops on
the Deer Isles, Maine
Naveed Davoodian1,2,*, Jason Bosworth1, and Nishanta Rajakaruna1
Abstract - Given the paucity of literature on plant-fungal interactions on serpentine soils
and limited investigation of serpentine geoecology in eastern North America, we examined
mycorrhizal colonization of Hypericum perforatum from adjacent serpentine and
granite outcrops on the Deer Isles, ME to determine whether plants were differentially
colonized based on substrate. We coincided our sampling with three phenologic stages
of H. perforatum (preflowering, flowering, postflowering) to determine possible differences
in colonization based on plant phenology. The levels of mycorrhizal colonization in
H. perforatum were not signifi cantly different between serpentine and granite sites, while
levels of colonization in postflowering plants were signifi cantly higher than in those at
preflowering and flowering stages.
Introduction
Serpentine soils are derived from igneous or metamorphic rocks consisting
of at least 70% ferromagnesian minerals (Kruckeberg 2002) and are generally
characterized by low levels of plant macronutrients; elevated levels of heavy
metals such as Ni, Cr, Cd, and Co; and Ca:Mg ratios of less than one (Brady et
al. 2005). Serpentine outcrops worldwide commonly display reduced plant productivity,
high rates of endemism, and vegetation markedly different from that
of surrounding areas (Whittaker 1954). For example, while only 1% of the state
of California is made up of serpentine rocks, 12.5% of the state’s endemic flora
is restricted to serpentine (Brady et al. 2005, Safford et al. 2005). Due to their
distinct characteristics and patchy distributions, both throughout the world and
on regional scales, serpentine outcrops are model settings for studies of ecology
and evolution (Boyd et al. 2009, Harrison and Rajakaruna 2011).
Much is known about vascular plants from serpentine habitats (Brady et al.
2005, Harrison and Rajakaruna 2011, Kruckeberg 2002), but the literature on
plant-fungal interactions on serpentine is limited (Branco and Ree 2010, Casper
and Castelli 2007, Doubková et al. 2012, Rajakaruna et al. 2009). Because of
the reduced availability of plant macronutrients (especially phosphorus) on serpentine
soils, plants on serpentine outcrops form mutualistic associations with
root symbionts, such as arbuscular mycorrhizal (AM) fungi, to overcome the
hardships presented by the soil (Strauss and Boyd 2011). AM fungi are members
of the fungal phylum Glomeromycota; they obligately associate with the
1College of the Atlantic, 105 Eden Street, Bar Harbor, ME 04609. 2New York Botanical
Garden, Institute of Systematic Botany, 2900 Southern Boulevard, Bronx, NY 10458-
5126. *Corresponding author - ndavoodian@coa.edu.
518 Northeastern Naturalist Vol. 19, No. 3
roots of angiosperms, gymnosperms, and pteridophytes, exchanging nutrients
with their hosts (Smith and Read 2008). They are widely distributed and are
major contributors to nutrient cycling in ecosystems (Read and Perez-Moreno
2003, Redecker 2008). Most higher plants participate in some kind of mycorrhizal
association, and plants that form mycorrhizal symbioses occur in twice as
many habitat types as those that do not form such associations, i.e., mycorrhizal
fungi appear to increase the niche widths of plants (Wilkinson and Dickinson
1995). Despite their ecological importance and ubiquity, few studies have
examined AM fungi in metal-enriched serpentine soils (Doherty et al. 2008,
Schechter and Bruns 2008). Given the varied and inconsonant data available on
the effects of toxic metals on AM symbioses (Pawlowska and Charvat 2004),
it is important to further investigate the status of these symbioses in metal-enriched
soils, particularly in light of the relevance of such studies to ecological
restoration efforts (O’Dell and Claassen 2009) and emerging bioremediation
technologies (Pilon-Smits and Freeman 2006).
While much effort has gone into elucidating the influence of ultramafi c
bedrock on associated organisms, such efforts in North America have largely
been carried out in the western portion of the continent, leaving the serpentine
geoecology of eastern North America largely unexplored (Boyd et al. 2009, Rajakaruna
et al. 2009). Ultramafi c rocks occur discontinuously along the eastern
edge of the Appalachian mountain range from Alabama to Newfoundland (Tyndall
and Hull 1999). For recent reviews of the serpentine geoecology of eastern
North America, see Alexander (2009) and Rajakaruna et al. (2009).
In this study, we examined the extent of mycorrhizal colonization between
specimens of Hypericum perforatum L. (Common St. John’s Wort; Hypericaceae)
collected from adjacent serpentine and granite outcrops on the Deer Isle
complex, ME to determine whether plants were differentially colonized by AM
fungi based on substrate. Furthermore, we compared different phenologic stages
of H. perforatum in order to determine any possible differences in mycorrhizal
colonization based on plant phenology. Previous research on these geologically
distinct sites revealed stark differences in assemblages of bryophytes (Briscoe et
al. 2009) and vascular plants (Pope et al. 2010). Furthermore, signifi cantly higher
levels of Mg, Fe, Ni, Zn, and Cd, as well as signifi cantly lower Ca:Mg ratios,
were documented in the serpentine soil compared to the granite soil, and signifi -
cantly higher Ni concentrations in H. perforatum growing on the serpentine site
was also shown (Pope et al. 2010).
Materials and Methods
Hypericum perforatum is a perennial that flowers from June to September
(Gleason and Cronquist 1991). In April, July, and October of 2009, a total of
89 specimens of Hypericum perforatum were collected at different phenologic
stages (preflowering, flowering, and postflowering). We defi ne preflowering as
plants exhibiting emerging young shoots in the spring, flowering as plants with
2012 N. Davoodian, J. Bosworth, and N. Rajakaruna 519
at least one flower in anthesis, and postflowering as plants senescing after the
flowering period. Plants were collected from Pine Hill and Settlement Quarry on
Little Deer Isle and Deer Isle, respectively, in Hancock County, ME. Pine Hill is
a former peridotite (serpentine) quarry on Little Deer Isle, and Settlement Quarry
is a former granite quarry on adjacent Deer Isle. For detailed descriptions and
histories of Pine Hill and Settlement Quarry, see Harris et al. (2007) and Briscoe
et al. (2009), respectively. For additional information on soil chemical and physical
features at these sites, see Pope et al. (2010).
At each site, collections were made randomly using a line transect. A compass
was placed roughly center in a collection area, and a transect was laid
along a randomly generated degree corresponding to a direction on the compass.
Plants were collected at predetermined, randomly generated intervals
along this transect. The transect was walked from end to end. This process was
repeated until at least 10 plants were collected for each unique combination of
effects (phenology × substrate).
Excess soil was manually cleaned off of roots and shipped in paper bags to Dr.
Efrén Cázares (Oregon State University, Corvallis, OR; www.mycoroots.com)
for assessment of AM colonization for each individual plant. The measurement
index used for colonization was percent colonization (length of root colonized by
hyphae, arbuscules, or vesicles over total length of root per plant).
Roots were cleared and stained through a method modifi ed from Phillips and
Hayman (1970). Roots were washed in running tap water and cut into segments to
fi t into glass vials where they were immersed in 10% KOH solution for 48 hours
at room temperature. Then the KOH solution was poured off, and the roots were
rinsed twice in tap water, placed in 1% HCl acid solution for 30 minutes, and then
rinsed in tap water afterward. Cleared samples were immersed for 12 hours at
room temperature in a staining solution of 0.5% trypan-blue (which stains fungal
wall cells) in lactoglycerol, rinsed with tap water, and stored in lactoglycerol or
water at 4 °C until examination. Stained roots were examined under a microscope
at 10–40x magnifi cation to determine presence or absence of mycorrhizal fungi.
Roots were placed in a Petri dish with a 1-cm grid marked on the bottom. Total
root length of each plant was estimated using the grid, and mycorrhizal colonization
was estimated by scanning all the roots under a microscope and recording
the presence of any hyphae, arbuscules, vesicles, or internal spores in each centimeter
of root.
Nonparametric tests were used to detect possible differences in the extent of
mycorrhizal colonization between sites and phenologic stages. Data were nonparametric,
even after multiple transformations. Mann-Whitney U-tests were
done to test for differences between sites (at each phenologic stage as well as
overall) and between phenologic stages (within sites) for mycorrhizal colonization
of H. perforatum. Software used for statistical analyses and fi gures were R
version 2.9.1 (Copyright © 2009 The R Foundation for Statistical Computing)
and MYSTAT version 12.02.00 (Copyright © 2007 SYSTAT Software, Inc.).
520 Northeastern Naturalist Vol. 19, No. 3
Results
Among all 89 H. perforatum plants collected, the root colonization varied
from 0% to 63%. The mean percent of root colonization for all plants collected
was 10% with a standard deviation of 13%, and most plants we observed had
colonization levels below the average (fig. 1).
There were no differences in root colonization levels between the serpentine
and granite sites (Mann-Whitney U-test P-values all > 0.25). However, within
each site, there was a signifi cant effect of phenology on colonization level; postfl
owering individuals exhibited signifi cantly higher colonization levels compared
to the other two stages, regardless of site (Mann-Whitney U-test P -values < 0.05;
fig. 2).
Discussion
Our results showed no signifi cant difference in the extent of AM colonization
in Hypericum perforatum between the serpentine and granite sites. Other studies
figure 1. Frequency histogram of AM colonization from 89 H. perforatum plants collected
in 2009.
2012 N. Davoodian, J. Bosworth, and N. Rajakaruna 521
have noted neutral, negative, and positive effects of various toxic elements (such
as those naturally occurring in serpentine areas) on different AM fungal species
(Bartolome-Esteban and Schenck 1994, Pawlowska and Charvat 2004, Smith and
Read 2008). Schechter and Bruns (2008) examined AM assemblages in ecotypes
of Collinsia sparsiflora fischer and C. Meyer (Spinster’s Blue-Eyed Mary; Scrophulariaceae)
on and off serpentine substrates in California and found that there
were no signifi cant differences in colonization between samples. These workers,
however, found that each ecotype was associated with a distinct AM fungal assemblage,
and they suggested that it is necessary for serpentine-adapted plants
to associate with serpentine-tolerant mycorrhizal fungal taxa in ultramafi c soils,
whether the plants are “choosing specifi c” fungi or “tapping nonspecifi cally”
into an edaphically influenced AM community. In contrast, in a study on the
serpentine-tolerant grass Avenula sulcata (J. Gay) Dumort, fitzsimons and Miller
figure 2. Box plot showing AM colonization for 89 H. perforatum plants. Y-axis displays
percent colonization. X-axis displays each unique pair of site and phenologic stage. The
bottom and top of a box show the fi rst and third quartiles, respectively. Bold lines show
medians. Bottom and top bars show minimum and maximum values, respectively, except
outliers, which are represented as open circles.
522 Northeastern Naturalist Vol. 19, No. 3
(2010) found no strong evidence of association with distinct AM communities
and concluded that plant adaptation to serpentine soils does not involve adapting
to unique microbial assemblages.
It is known that exposing arbuscular mycorrhizae to increasing metal concentrations,
regardless of whether the fungal strains involved are sensitive or
resistant to toxic metals, usually reduces the percentage of root colonization,
though populations of fungi isolated from metal-laden environments are often
more metal-resistant (Meharg and Cairney 2000). In a review of the available
literature, Meharg and Cairney (2000) concluded that AM fungi provide little
to no enhanced metal resistance to their hosts. Furthermore, these workers suggested
that AM associations in metal-laden environments are not maintained
because they confer metal resistance to the plant host but rather for the same
reasons they are maintained in other environments, namely improved nutrient
acquisition and water relations. They further suggest that AM fungi and their
hosts have co-evolved to survive in metal-enriched environments and thus can
exploit extremely metalliferous ecological niches. This co-evolution, coupled
with evidence that the evolution of metal resistance in AM fungi can be rapid
(Meharg and Cairney 2000), could possibly explain why our investigation found
no difference in colonization between the serpentine and granite sites despite
the fact that increased metal concentrations are known to decrease colonization
levels. Similarly, Gonçalves et al. (2001), fi nding that Ni concentration at a serpentine
site in Portugal did not affect the extent of AM colonization in Festuca
brigantina (Markgr.-Dann.) Markgr.-Dann., suggested that the fungi are adapted
to serpentine soil. In contrast to the conclusion reached by Meharg and Cairney
(2000), at least one study has shown that AM fungi can reduce the toxic effects
of metals on their host plants (Joner and Leyval 1997).
Throughout our study, the values we observed for percent root colonization
were fairly low, as two-thirds of our samples exhibited between 0–10% mycorrhizal
colonization (fig. 1). By contrast, Hopkins (1987), studying 27 species in
a serpentine grassland in California in May, found high levels of colonization:
91.6% of the herbaceous cover sampled exhibited over 75% mycorrhizal colonization,
and 97.7% of the herbaceous cover exhibited over 50% colonization. In
24 samples of Collinsia sparsiflora taken in March from serpentine and nonserpentine
sites in California, Schechter and Bruns (2008) found all samples to be
highly colonized (44–57%), whereas our 89 H. perforatum plants had a mean of
10%, with only 3 plants exhibiting colonization levels equal to or greater than
40%. Hypericum perforatum is native to Europe, North Africa, and Asia (Maron
et al. 2004) but not to North America (Haines and Vining 1998). There is evidence
that the species has been introduced into North America multiple times
(Maron et al. 2004). Populations of the plant in North America invest less in
root biomass and more in fi rst-year reproduction than populations in the native
range, suggesting a shift towards a more annual, weedy life history compared to
conspecifi cs in the native range―a pattern observed in many introduced species
(Seifert et al. 2009). Furthermore, there is strong evidence that North American
2012 N. Davoodian, J. Bosworth, and N. Rajakaruna 523
populations of H. perforatum have evolved reduced dependence on mycorrhizal
fungi (Seifert et al. 2009). Several introduced species have been found to be less
dependent on AM fungi than native species with which they co-occur (Seifert
et al. 2009, Vogelsang and Bever 2009). Seifert et al. (2009) speculated on the
selective pressures involved in causing this phenomenon in H. perforatum and
non-native species in general, and suggested that anthropogenic disturbance that
disrupts and degrades natural AM fungal communities (e.g., intensive agriculture)
could select for plants with reduced mycorrhizal dependence. Hypericum
perforatum’s reduced mycorrhizal dependence in North America could explain
the low root colonization values we observed.
Our results show signifi cantly higher colonization in the postflowering stage
(October) than in the preflowering and flowering stages (April and July). Phenology
appears to have a strong effect on patterns of AM colonization. A study of
mycorrhizae in prairie tallgrasses found colonization to be greatest in late summer
or fall, and it was suggested that the mycorrhizal fungi involved might be
parasitic at that time (Bentivenga and Hetrick 1992, Kennedy et al. 2002). This
possibility could be true for our observations, especially in the context of reduced
mycorrhizal dependence of H. perforatum in North America (Seifert et al. 2009).
Thus, the greater colonization we observed in postflowering collections could
represent fungal symbionts opportunistically parasitizing their plant hosts as the
plants age.
It appears that mycorrhizal colonization in H. perforatum is lower on Pine Hill
(serpentine) than on Settlement Quarry (granite), because mean colonization is
higher on Settlement Quarry in the preflowering and postflowering stages. Our
results, however, indicate that the effect of substrate is minor, while the effect of
phenology is signifi cant. It is possible that in this case plant phenology is a proxy
for other seasonal variables, such as temperature and/or soil moisture. If plant
phenology is the “actual” effect, however, it implies that investigators comparing
mycorrhizae from different substrates should take particular care in noting
whether phenology is aligned between samples. Otherwise, differences that are
due to variations in plant phenology among sites could be mistakenly interpreted
as being due to edaphic effects.
Acknowledgments
This work was made possible by generous support from the US Environmental Protection
Agency (GRO Fellowship for Undergraduate Environmental Study, F9P11071) and
College of the Atlantic (Rothschild Student-Faculty Collaborative Research Grant). The
guidance and expertise of Dr. Thomas J. Volk at the University of Wisconsin-La Crosse
was instrumental in the early stages of this research. We are especially thankful to Dr.
Chris Petersen at College of the Atlantic, who greatly assisted with research design and
data analysis, Dr. Efrén Cázares/MycoRoots at Oregon State University for assessing our
samples and providing us with his expert opinion, Dr. Don Cass at College of the Atlantic
for generously allowing our extended use of his space and supplies, and Tanner Harris and
two anonymous reviewers for helpful comments and edits of an earlier version of the manuscript.
This paper is based on the fi nal undergraduate thesis of the fi rst-named author.
524 Northeastern Naturalist Vol. 19, No. 3
Literature Cited
Alexander, E.B. 2009. Serpentine geoecology of the eastern and southeastern margins of
North America. Northeastern Naturalist 16 (Special Issue 5):223–252.
Bartolome-Esteban, H., and N.C. Schenck. 1994. Spore germination and hyphal growth
of arbuscular mycorrhizal fungi in relation to soil aluminum saturation. Mycologia
86:217–226.
Bentivenga, S.P., and B.A.D. Hetrick. 1992. Seasonal and temperature effects on mycorrhizal
activity and dependence of cool- and warm-season tallgrass prairie grasses.
Canadian Journal of Botany 70:1596–1602.
Boyd, R.S., A.R. Kruckeberg, and N. Rajakaruna. 2009. Biology of ultramafi c rocks
and soils: Research goals for the future. Northeastern Naturalist 16 (Special Issue
5):422–440.
Brady, K.U., A.R. Kruckeberg, and H.D. Bradshaw, Jr. 2005. Evolutionary ecology of
plant adaptation to serpentine soils. Annual Review of Ecology, Evolution, and Systematics
36:243–266.
Branco, S., and R.H. Ree. 2010. Serpentine soils do not limit mycorrhizal fungal diversity.
PloS ONE 5:e11757.
Briscoe, L.R.E., T.B. Harris, E. Dannenberg, W. Broussard, F.C. Olday, and N. Rajakaruna.
2009. Bryophytes of adjacent serpentine and granite outcrops on the Deer Isles,
Maine, USA. Rhodora 111:1–20.
Casper, B.B., and J.P. Castelli. 2007. Evaluating plant-soil feedback together with competition
in a serpentine grassland. Ecology Letters 10:394–400.
Doherty, J.H., B.M. Ji, and B.B. Casper. 2008. Testing nickel tolerance of Sorghastrum
nutans and its associated soil microbial community from serpentine and prairie soils.
Environmental Pollution 151:593–598.
Doubková, P, J. Sudaa, and R. Sudováa. 2012. The symbiosis with arbuscular mycorrhizal
fungi contributes to plant tolerance to serpentine edaphic stress. Soil Biology
and Biochemistry 44: 56–64.
fitzsimons, M.S., and R.M. Miller. 2010. Serpentine soil has little influence on the rootassociated
microbial community composition of the serpentine-tolerant grass species
Avenula sulcata. Plant and Soil 330:395–405.
Gleason, H.A., and A. Cronquist. 1991. Manual of Vascular Plants of Northeastern
United States and Adjacent Canada. 2nd Edition. The New York Botanical Garden,
Bronx, NY.
Gonçalves, S.C., M. A. Martins-Loução, and H. Freitas. 2001. Arbuscular mycorrhizas
(AM) of Festuca brigantina, an endemic serpentinophyte from Portugal. South African
Journal of Science 97:571–572.
Haines, A., and T.F. Vining. 1998. Flora of Maine: A Manual for Identifi cation of Native
and Naturalized Vascular Plants of Maine. V.F. Thomas Company, Bar Harbor, ME.
Harris, T.B., F.C. Olday, and N. Rajakaruna. 2007. Lichens of Pine Hill, a peridotite
outcrop in Eastern North America. Rhodora 109:430–447.
Harrison, S.P., and N. Rajakaruna. 2011. What have we learned from serpentine about
evolution, ecology, and other sciences? Pp. 417–428, In S.P. Harrison and N. Rajakaruna
(Eds.). Serpentine: The Evolution and Ecology of a Model System. University
of California Press, Berkeley, CA.
Hopkins, N.A. 1987. Mycorrhizae in a California serpentine grassland community. Canadian
Journal of Botany 65:484–487.
2012 N. Davoodian, J. Bosworth, and N. Rajakaruna 525
Joner, E.J., and C. Leyval. 1997. Uptake of 109Cd by roots and hyphae of a Glomus mosseae/
Trifolium subterraneum mycorrhiza from soil amended with high and low concentrations
of cadmium. New Phytologist 135:353–360.
Kennedy, L.J., R.L. Tiller, and J.C. Stutz. 2002. Associations between arbuscular mycorrhizal
fungi and Sporobolus wrightii in riparian habitats in arid southwestern North
America. Journal of Arid Environments 50:459–475.
Kruckeberg, A.R. 2002. Geology and Plant Life: The Effects of Landforms and Rock
Type on Plants. University of Washington Press, Seattle, WA.
Maron, J.L., M. Vilà, R. Bommarco, S. Elmendorf, and P. Beardsley. 2004. Rapid evolution
of an invasive plant. Ecological Monographs 74:261–280.
Meharg, A.A., and J.W.G. Cairney. 2000. Co-evolution of mycorrhizal symbionts and
their hosts to metal-contaminated environments. Pp. 69–112, In A.H. fitter and D.G.
Raffaelli (Eds.). Advances in Ecological Research. 30. Academic Press, London.,UK
O’Dell, R.E., and V.P. Claassen. 2009. Serpentine revegetation: A review. Northeastern
Naturalist 16 (Special Issue 5):253–271.
Pawlowska, T.E., and I. Charvat. 2004. Heavy metal stress and developmental patterns
in arbuscular mycorrhizal fungi. Applied and Environmental Microbiology
70:6643–6649.
Phillips, J.M., and D.S. Hayman. 1970. Improved procedure for clearing roots and staining
parasitic and vesicular-arbuscular mycorrhizal fungi for rapid assessment of infection.
Transactions of the British Mycological Society 55:158–161.
Pilon-Smits, E.A.H., and J.L. Freeman. 2006. Environmental cleanup using plants: Biotechnological
advances and ecological considerations. Frontiers in Ecology and the
Environment 4:203–210.
Pope, N., T.B. Harris, and N. Rajakaruna. 2010. Vascular plants of adjacent serpentine
and granite outcrops on the Deer Isles, Maine, USA. Rhodora 112:105–141.
Rajakaruna, N., T.B. Harris, and E.B. Alexander. 2009. Serpentine geoecology of
eastern North America: A review. Rhodora 111:21–108.
Read, D.J., and J. Perez-Moreno. 2003. Mycorrhizas and nutrient cycling in ecosystems:
A journey towards relevance? New Phytologist 157:475–492.
Redecker, D. 2008. Glomeromycota. Arbuscular mycorrhizal fungi and their
relative(s). Version 14 January 2008. Available online at http://tolweb.org/
Glomeromycota/28715/2008.01.14. Accessed 20 January 2008.
Safford, H.D., J.H. Viers, and S.P. Harrison. 2005. Serpentine endemism in the California
flora: A database of serpentine affi nity. Madroño 52:222–257.
Schechter, S.P., and T.D. Bruns. 2008. Serpentine and non-serpentine ecotypes of Collinsia
sparsiflora associate with distinct arbuscular mycorrhizal fungal assemblages.
Molecular Ecology 17:3198–3210.
Seifert, E.K., J.D. Bever, and J.L. Maron. 2009. Evidence for the evolution of reduced
mycorrhizal dependence during plant invasion. Ecology 90:1055–1062.
Smith, S.E., and D.J. Read. 2008. Mycorrhizal Symbiosis. 3rd Edition. Academic Press,
London, UK.
Strauss, S.Y., and R.S. Boyd. 2011. Herbivory and other cross-kingdom interactions on
harsh soils. Pp. 181–200, In S.P. Harrison, and N. Rajakaruna (Eds.). Serpentine:
The Evolution and Ecology of a Model System. University of California Press,
Berkeley, CA.
526 Northeastern Naturalist Vol. 19, No. 3
Tyndall, R.W., and J.C. Hull. 1999. Vegetation, flora, and plant physiological ecology of
serpentine barrens of eastern North America. In R.C. Anderson, J.S. Fralish, and J.M.
Baskin (Eds.). Savannas, Barrens, and Rock Outcrop Plant Communities of North
America. Cambridge University Press, New York, NY.
Vogelsang, K.M., and J.D. Bever. 2009. Mycorrhizal densities decline in association with
nonnative plants and contribute to plant invasion. Ecology 90:399–407.
Whittaker, R.H. 1954. The ecology of serpentine soils. IV. The vegetation response to
serpentine soils. Ecology 35:275–288.
Wilkinson, D.M., and N.M. Dickinson. 1995. Metal resistance in trees: The role of mycorrhizae.
Oikos 72:298–300.