Relationships of Modeled Nitrogen Loads with Marsh Fish
in the Narragansett Bay Estuary, Rhode Island
Cathleen Wigand, Heather Smith, Cassius Spears, Brandon Keith,
Richard McKinney, Marnita Chintala, and Kenneth Raposae
Northeastern Naturalist, Volume 22, Issue 1 (2015): 1–9
Full-text pdf (Accessible only to subscribers. To subscribe click here.)

Access Journal Content
Open access browsing of table of contents and abstract pages. Full text pdfs available for download for subscribers.
Current Issue: Vol. 30 (3)
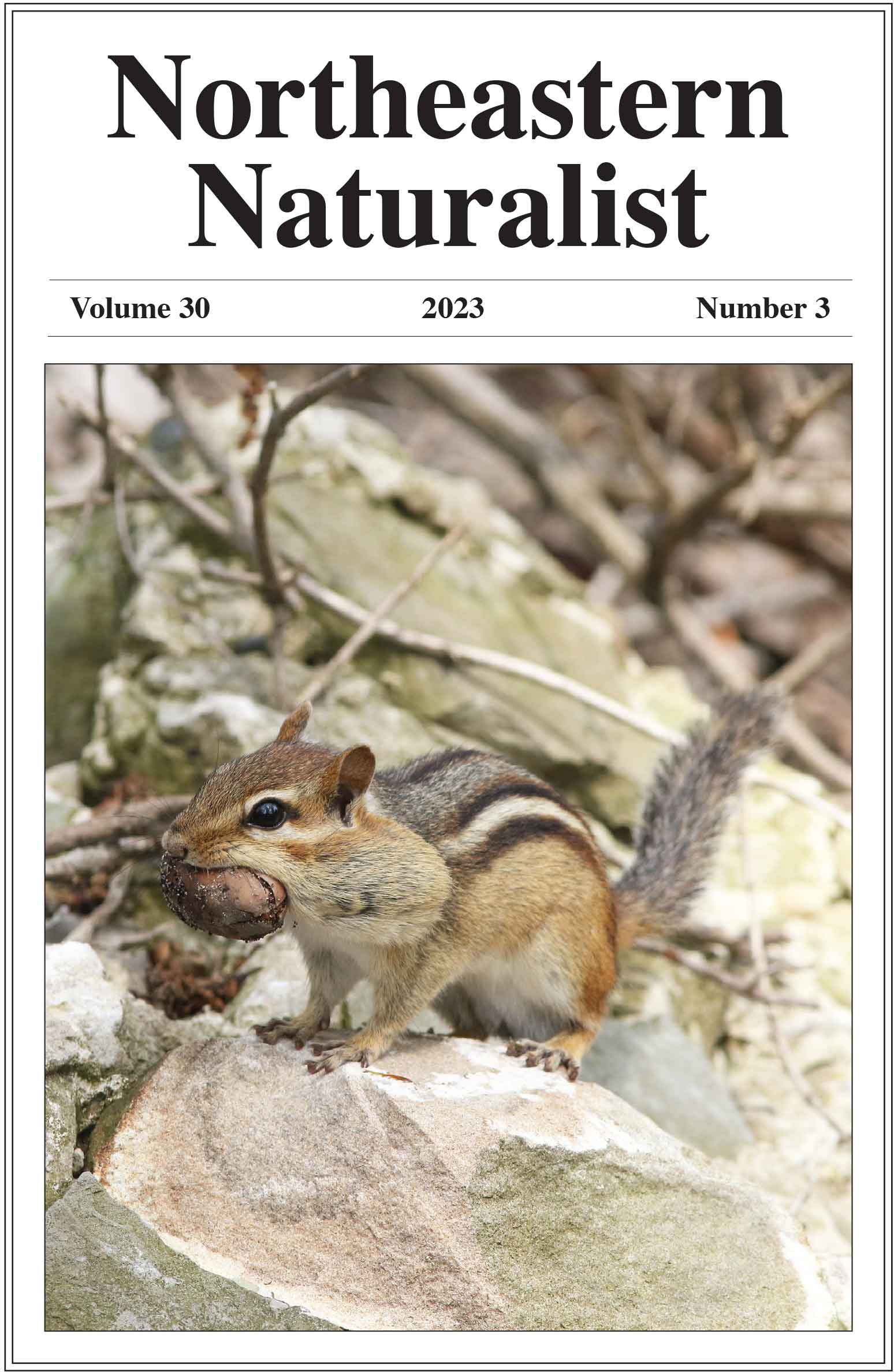
Check out NENA's latest Monograph:
Monograph 22
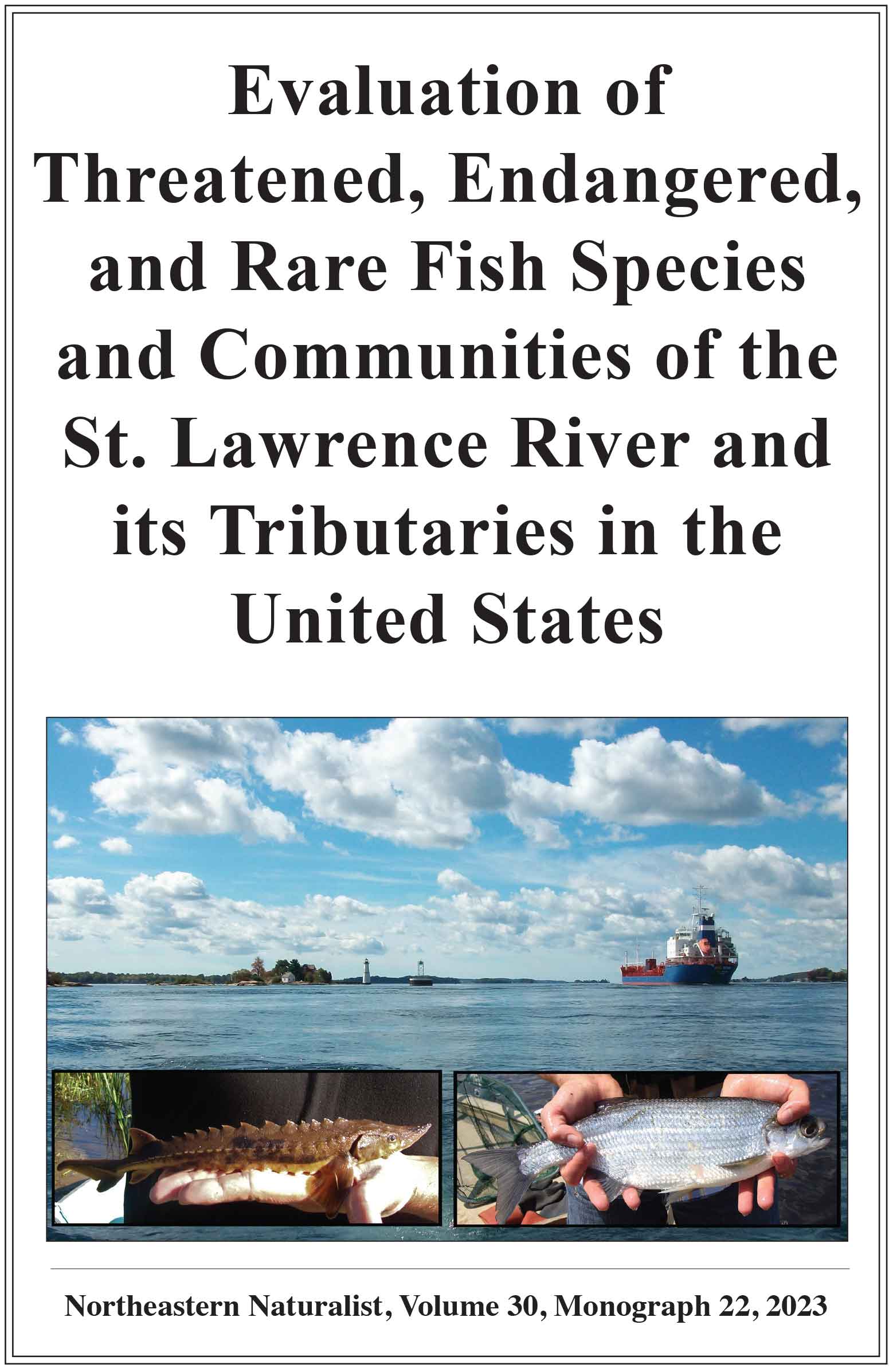
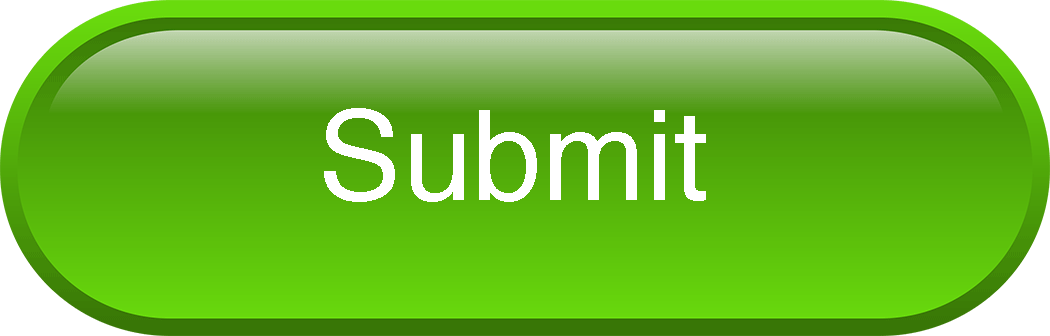
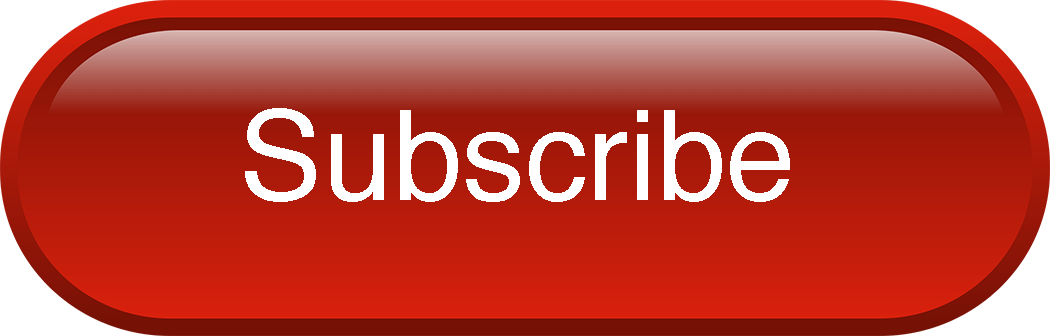
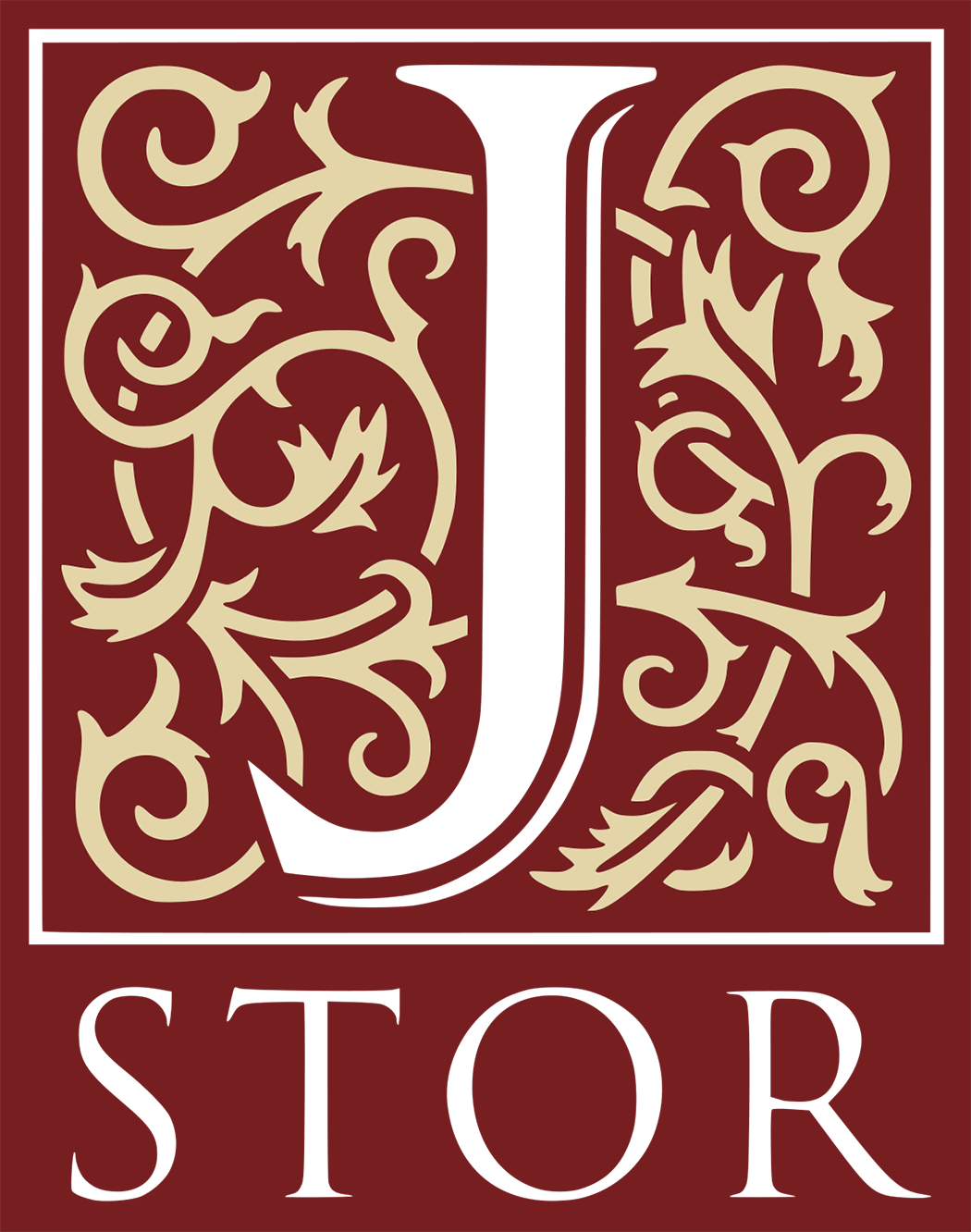
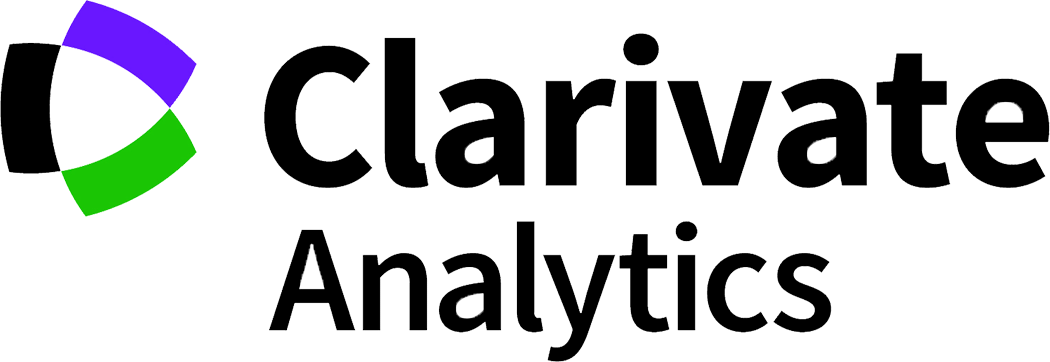
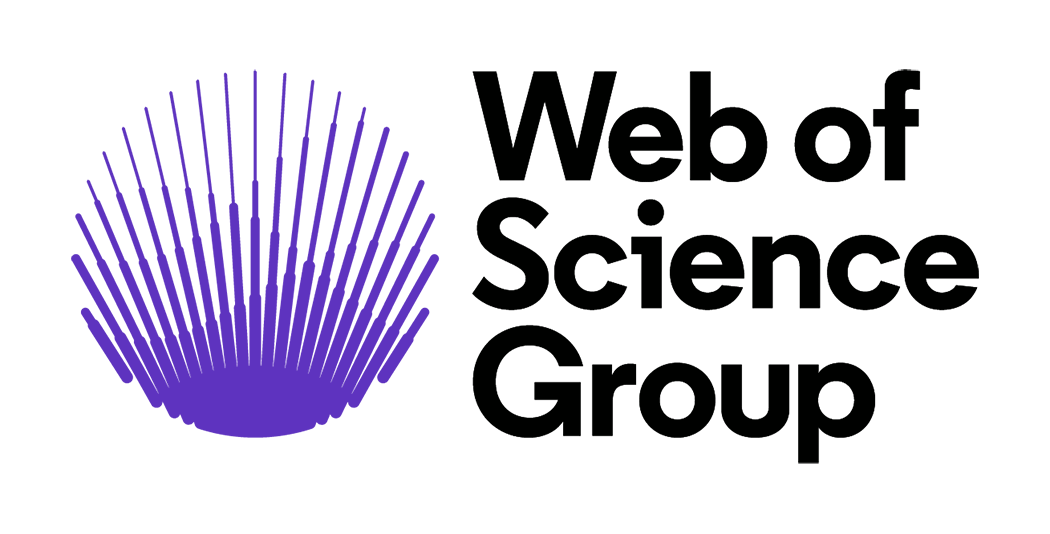
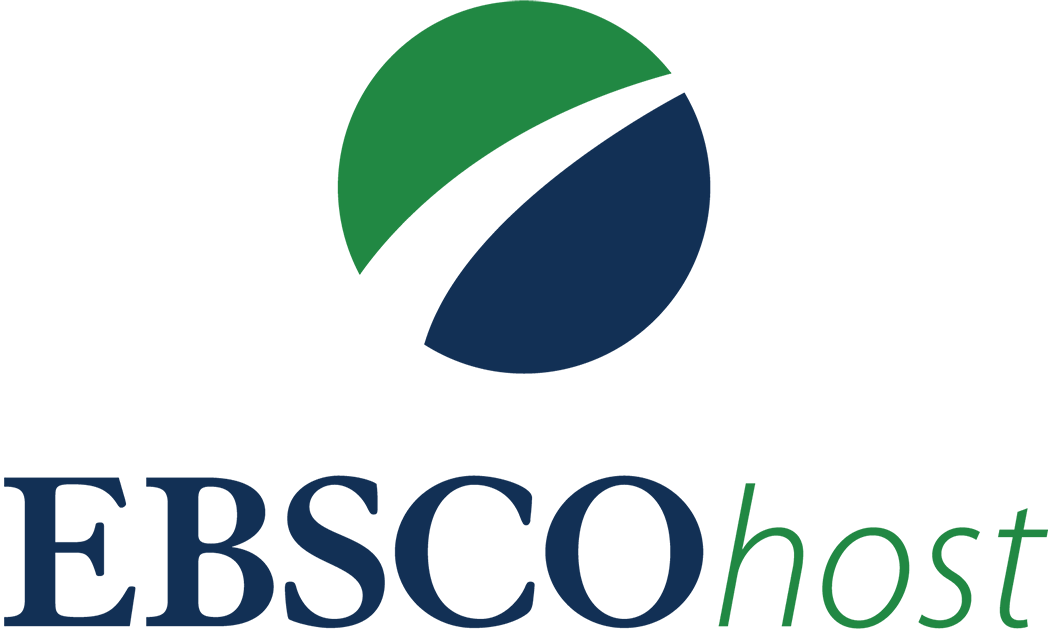
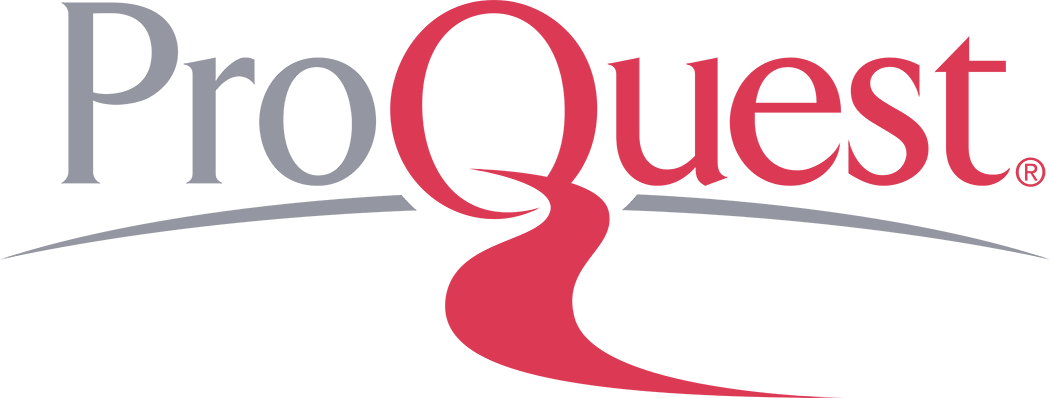
Northeastern Naturalist Vol. 22, No. 1
C. Wigand, H. Smith, C. Spears, B. Keith, R. McKinney, M. Chintala, and K. Raposa
2015
1
2015 NORTHEASTERN NATURALIST 22(1):1–9
Relationships of Modeled Nitrogen Loads with Marsh Fish
in the Narragansett Bay Estuary, Rhode Island
Cathleen Wigand1,*, Heather Smith1, Cassius Spears1, Brandon Keith1,
Richard McKinney1, Marnita Chintala1, and Kenneth Raposa2
Abstract - The human population and associated watershed development has risen steadily
since the 1850s in Rhode Island. With these increases, human-derived wastewater has also
risen dramatically, resulting in increasing nitrogen loads to estuarine systems. In this study,
we examined relationships of modeled watershed nitrogen loads of 6 coastal subwatersheds
of varying land development with the stable nitrogen isotope ratio (δ15N) of salt marsh fish
and larvae. There was a significant positive relationship (r = +0.97, P < 0.05) between the
watershed modeled percent wastewater and δ15N in Fundulus heteroclitus L. (Common
Mummichog), and significantly higher (P < 0.05) δ15N in fish larvae collected from developed
mainland marsh sites compared to less-developed island marsh sites. Our results
support earlier published findings that fish in coastal marshes are assimilating nitrogen
derived from watershed wastewater sources. Furthermore, there was an inverse relationship
(P = 0.05) between the modeled percentage of human wastewater and mummichog size. The
increasing loads of watershed nitrogen entering into coastal salt marshes are a concern because
it is unclear how well salt marsh ecosystems can continue to assimilate high nitrogen
inputs especially when also subjected to a warming climate.
Introduction
Fish in salt marshes are known to be hardy and opportunistic since they are
adapted to a life in often harsh conditions (Bigelow and Schroeder 1953). Salt marsh
fish live in water with rapidly changing salt and tide levels and, in recent times,
increasing loads of anthropogenic nutrients. The human population in Rhode Island
has risen steadily since the 1850s, and with this increase, human-derived wastewater
has also risen dramatically (Nixon et al. 2008). While some studies report that
increased nutrient inputs into coastal habitats may increase primary production and
food resources available for some coastal marsh fish (Nixon 1992, Oczkowski et
al. 2009, Tober et al. 2000), others report adverse effects of high nutrient loading
due to low-oxygen events, sometimes even resulting in fishkills and mass mortality
of marine invertebrates (Deacutis 2008; Diaz and Rosenberg 1995, 2001). Longterm
nutrient loadings have also been implicated in marsh loss (Deegan et al. 2012,
Wigand et al. 2014). Researchers have found direct relationships between the rise
in the human population, coastal watershed land development, and the stable nitrogen
isotope ratios of (δ15N) in Spartina alterniflora, Loisel (Smooth Cordgrass),
1USEPA, National Health and Environmental Effects Research Laboratory, Atlantic Ecology
Division, Narragansett, RI 02882. 2Narragansett Bay National Estuarine Research
Reserve, 55 South Reserve Drive, Prudence Island, RI 02872. *Corresponding author -
Wigand.Cathleen@epa.gov.
Manuscript Editor: Hunter J. Carrick
Northeastern Naturalist
2
C. Wigand, H. Smith, C. Spears, B. Keith, R. McKinney, M. Chintala, and K. Raposa
2015 Vol. 22, No. 1
Geukensia demissa, Dillwyn (Ribbed Mussel), and Fundulus heteroclitus, L. (Common
Mummichog) in tidal wetlands (e.g., Bannon and Roman 2008, Cole et al.
2004, McClelland and Valiela 1998, McKinney et al. 2001). These relationships
may result from elevated δ15N values characteristic of human-derived wastewater.
In this study, we examined the stable nitrogen isotope ratios, abundance, and size of
small fish in 6 subwatersheds of the Narragansett Bay Estuary (RI) along a gradient
of high to low nitrogen loadings. In addition, the δ15N of larval fish were measured
from 3 developed mainland sites and 3 less-developed island sites to see if there was
an anthropogenic signal in the larvae from the developed watersheds. We examine
and report the relationships of the fish δ15N, size, and abundance with the modeled
watershed nitrogen loadings.
Site Description and Methods.
Marsh fish were sampled twice at each site in the summer of 2005 in 6 Narragansett
Bay subestuaries, which had varying watershed land use and estimated nitrogen
loads (Table 1, Fig. 1). Seines were cast from shore (i.e., quarter circle haul) during
an ebb tide using a 6-m-long seine (3-mm mesh). Larval fish were collected by pit
traps (plexiglass trays: 28 x 18 x 4 cm) set flush with the soil surface in the high
marsh (mixed meadow of Spartina patens (Aiton) Muhl [Saltmeadow Cordgrass]
and Distichlis spicata (L.) Greene [Saltgrass]) at 3 developed mainland sites (Bissel
Cove, Old Mill Cove, and Passeonkquis Cove) and at 3 less-developed coastal
island sites (Fox Hill Pond, Jenny Marsh, and Providence Point) (Fig. 1). Pit traps
were placed in 2 parallel lines of 5 traps each, a few meters apart, coincident with
flooding of the high marsh at each site. The pit traps were sampled on spring tides
around the full and new moons on 7 dates between 21 June–17 September.
Estimates of the watershed nitrogen loads entering the salt marshes were
previously reported (Wigand et al. 2003) and calculated using (1) 1995 aerial
photography (1:24,000 scale) showing land use and land cover and (2) a landbased
nutrient loading model (Valiela et al. 1997). The nutrient-loading model
(NLM: NLOAD homepage, http://nload.mbl.edu) was developed and verified for
Table 1. Land use and modeled nitrogen loads for six Narragansett Bay subwatersheds. Ag. = agricultural;
Ind. and comm. = industrial and commercial; Recr. = recreational; Natural lands include inland
wetlands, forests, and brush lands; and Resid. = residential. Site locations are shown in Figure 1: Jenny
Marsh (JEN), Sapowet (SAP), Mary Donovan (DON), Bissel Cove (BIS), Watchemoket (WAT), and
Apponaug Cove (APP).
% land use and land cover Modeled watershed nitrogen loads
Ind. and Natural Nitrogen load % wastewater
Location Ag. comm. Recr. lands Resid. (Kg N ha-1 y-1) nitrogen
Jenny Marsh 0.0 0.0 0.0 81.3 4.0 2.5 20.3
Sapowet 34.7 0.4 0.1 49.0 13.8 183.3 21.7
Mary Donovan 20.0 0.5 0.1 57.2 10.0 412.6 25.7
Bissel Cove 4.7 1.9 1.2 55.7 22.1 3183.1 68.5
Watchemoket 0.0 9.5 13.9 3.8 56.0 6727.0 82.0
Apponaug Cove 3.1 12.3 3.8 22.7 43.3 11407.2 83.3
Northeastern Naturalist Vol. 22, No. 1
C. Wigand, H. Smith, C. Spears, B. Keith, R. McKinney, M. Chintala, and K. Raposa
2015
3
Cape Cod, MA (Valiela et al. 1997, 2000) and has additionally been verified for
Barnegat Bay, NJ (Bowen et al. 2007a, b). Each of the components required to estimate
the nitrogen loading in the NLM are subject to uncertainty, and Collins et
Figure 1. Location of subwatersheds (n = 6) used to model nitrogen loadings, and locations
of mainland (n = 3) and island (n = 3) pit-trap sites where we sampled fish larvae. We cast
seines in open water in marshes adjacent to the 6 subwatersheds.
Northeastern Naturalist
4
C. Wigand, H. Smith, C. Spears, B. Keith, R. McKinney, M. Chintala, and K. Raposa
2015 Vol. 22, No. 1
al. (2000) calculated a 13% uncertainty in the loading estimates of the NLM using
a bootstrap resampling method. The NLM has more recently been used to successfully
estimate nitrogen loading to 74 subwatersheds in southern New England
(Latimer and Charpentier 2010) and 33 subwatersheds of the Great South Bay, NY
(Kinney and Valiela 2011). The NLM estimates nitrogen loads from fertilizer application,
human wastewater, and atmospheric deposition to 4 land-use types (i.e.,
natural vegetation, turf, agricultural land, and impervious surface). The model
accounts for attenuation as the nitrogen passes through the watershed surface and
subsurface zones and calculates the net input of nitrogen as the sum of the inputs
minus losses in various land-use types. The estimate of the nitrogen load to the
marsh is then calculated as the sum of the net loads determined from the NLM
from fertilizer, human wastewater, and atmospheric deposition,
We randomly selected 10 seine-collected mummichogs from each site and froze
them for δ15N analysis. We removed their guts and heads, dried the remaining portions,
and ground and homogenized them with a mortar and pestle. We treated larval
samples similarly except we did not remove their guts and heads. We used continuous-
flow isotope-ratio mass spectrometry to measure the δ15N of the samples, and
known differences in nitrogen isotope ratios to examine if there was an anthropogenic
nitrogen signal in the fish samples. Compared with estimates of atmospheric
deposition of nitrogen (δ15N of +2 to +8 ‰, Kreitler and Jones 1975) and fertilizer
(δ15N of -3 to +3 ‰; Freyer and Aly 1974, Macko and Ostrom 1994), nitrogen derived
from wastewater (δ15N of +10 to +22 ‰; Aravena et al. 1993, Kreitler and Jones
1975) is relatively enriched in 15N and has a higher stable nitrogen isotope ratio.
Therefore, human-derived nitrogen inputs would result in an enriched δ15N in fish
when the percentage of watershed wastewater increases.
Results and Discussion.
We found a significant relationship (r = +0.97, P < 0.05) between the watershed
modeled wastewater nitrogen loads (Table 1) and the δ15N in Common Mummichog
(Fig. 2). The varying δ15N of the fish collected from 6 subestuaries in Narragansett
Bay having high to low watershed nitrogen loads (Table 2) support the premise
proposed by others (e.g., Cole et al. 2004, McClelland and Valiela 1998) that watershed
nitrogen was entering into salt marshes and being assimilated by biota. The
larval fish collected from the developed mainland sites had significantly higher
δ15N (13.2 ± 1.3 ‰) than those collected from the less-developed island marshes
(9.9 ± 0.16 ‰) (P < 0.05, using a two-tailed t-test on log transformed data), further
supporting the hypothesis that the watershed loads of human-derived nitrogen from
developed areas are being assimilated by marsh fish. In contrast, Pruell et al. (2006)
inferred that the changes in stable nitrogen isotope ratio in biota among 3 Narragansett
Bay subestuaries might be due to the differences in distance from point sources
of sewage outfall from the head of the bay.
Previously, the stable nitrogen isotope ratios of Smooth Cordgrass (Wigand
et al. 2001) and Ribbed Mussels (McKinney et al. 2001) were measured in 10
Narragansett Bay salt marshes. Similar to the relationship we found with Common
Northeastern Naturalist Vol. 22, No. 1
C. Wigand, H. Smith, C. Spears, B. Keith, R. McKinney, M. Chintala, and K. Raposa
2015
5
Figure 2. Relationships of the stable nitrogen isotope ratios of Common Mummichog,
Ribbed Mussel, and Smooth Cordgrass with the wastewater fraction of the modeled nitrogen
loads. The Ribbed Mussel and Smooth Cordgrass results were first reported in McKinney et
al. (2001) and Wigand et al. (2001), respectively.
Table 2. The stable nitrogen isotope ratios (δ15N), abundance, and size of Commmon Mummichogs
from 6 subestuaries with varying modeled watershed nitrogen loads. Location of sites are shown in
Figure 1, and watershed nitrogen loads are provided in Table 1.
Site δ15NA Abundance (total #)B Size (mm)C
Jenny Marsh 9.1 161.5 63.9
Sapowet 9.0 81.0 57.8
Mary Donovan Marsh 9.2 101.0 44.3
Bissel Cove 11.8 88.5 29.1
Watchemoket Cove 14.7 21.5 33.0
Apponaug Cove 13.7 421.5 41.7
Among-site mean 11.3 145.8 45.0
Among-site SE 1.0 58.1 5.6
ACommon Mummichog stable nitrogen isotope ratio from sample of 1 0 fish.
BAverage # mummichogs in 2 seine catches.
CAverage size of 10 mummichogs.
Northeastern Naturalist
6
C. Wigand, H. Smith, C. Spears, B. Keith, R. McKinney, M. Chintala, and K. Raposa
2015 Vol. 22, No. 1
Mummichog in this study, the stable nitrogen isotopic ratios of Smooth Cordgrass
and Ribbed Mussels also significantly increased with increasing percent wastewater
nitrogen loads estimated from the watershed NLM model. When the δ15N of all 3
organisms among the Narragansett Bay sites are considered together, it is apparent
that the stable nitrogen isotope ratios of the Common Mummichog and Ribbed
Mussel are higher than the Smooth Cordgrass ratios (Fig. 2). Since stable nitrogen
isotopic ratios increase in species further up the food chain, it is not a surprise
that the fish and mussels would have a higher signal than the plants at the base of
the food chain. The fish are omnivores, and their stable nitrogen isotope ratios are
elevated because of the consumption of small mollusks and crustaceans in addition
to plant detritus. Ribbed Mussels are filter-feeders and consume plant detritus,
phytoplankton, bacteria, and other particulate matter. McClelland et al. (1997) demonstrated
trophic transfer using stable nitrogen isotope ratios in biota in 2 Waquoit
Bay, MA, sites (i.e., Sage Lot Pond, Childs River).
In the seines, we primarily caught juvenile Common Mummichog, but there
were also small numbers of Menidia menidia, L. (Atlantic Silverside) and Cyprinidon
variegatus, Lacépède (Sheepshead Minnow). In addition to fish species, the
seines also captured small shrimp, crabs, and Ulva lactuca, L. (Sea Lettuce). The
most (422) and least (22) numbers of mummichogs were collected from the 2 most
urbanized sites with the greatest modeled wastewater nitrogen loads, Apponaug
Cove and Watchemoket Cove, respectively. Average mummichog size among the
marsh sites was 45 ± 6 mm, and average abundance among sites was 146 ± 58 fish
(Table 2). There was an inverse trend (r = -0.81, P = 0.05) between the watershed
modeled percent wastewater nitrogen and mummichog size, and a positive trend
(r = +0.73, P = 0.10) of the watershed modeled total nitrogen load and mummichog
abundance in Narragansett Bay. LaBrecque et al. (1996) and Tober et al. (2000)
reported significantly greater abundances but no difference in growth rates of Common
Mummichogs in subestuaries with greater nitrogen loadings in Waquoit Bay,
MA. Our seining study was of short duration and only 6 marshes in Narragansett
Bay were sampled, so we propose that future long-term and more detailed studies
might better elucidate the relationships of fish abundance, size, and growth with
watershed nitrogen loadings in a number of different urban estuaries.
Urbanization and the associated cultural eutrophication (especially the overenrichment
of nitrogen) is known to cause reduced system oxygen levels and
macrofauna declines (Deacutis 20008; Diaz and Rosenberg 1995, 2001), plant species
shifts on the salt marsh landscape (Bertness et al. 2002, Silliman and Bertness
2004, Wigand et al. 2003), and marsh-bank deterioration (Deegan et al. 2012). The
increasing loads of watershed nitrogen entering into New England salt marshes
are a concern, because it is unclear how well salt marsh ecosystems and fish in
particular can assimilate these inputs, especially when also subjected to the additional
stress of a warming climate (Nixon et al. 2004). Because salt marshes are
located between the coastal sea and the uplands, and provide numerous ecosystem
services, such as fish habitat, flood abatement, and water-quality maintenance, it is
important to monitor and understand watershed nitrogen effects on coastal systems.
Northeastern Naturalist Vol. 22, No. 1
C. Wigand, H. Smith, C. Spears, B. Keith, R. McKinney, M. Chintala, and K. Raposa
2015
7
Watershed nutrient sources are likely some of the most appropriate for managing
estuarine water-quality conditions because these sources are most amenable to
watershed-scale remedial action (Latimer and Charpentier 2010).
Acknowledgments
Heather Smith, Cassius Spears, and Brandon Keith were sponsored through the EPA/
University of Rhode Island Environmental Sciences Research and Training Opportunities
Program (RTOP), a multi-institutional cooperative agreement (CT8 3045). During the summer
of 2005, they conducted research with mentor scientists, Cathleen Wigand, Richard
McKinney, and Marnita Chintala of the US Environmental Protection Agency, Narragansett
Laboratory. Kenneth Raposa is the Research Coordinator at the Narragansett Bay National
Estuarine Research Reserve, where 2 of our sampling sites were located. Special thanks to
Kim Anderson and Darryl Keith for coordinating RTOP and connecting the students with
the EPA mentors. We are also grateful to the GIS expertise of Mike Charpentier in determining
the land use for the watersheds described in this study. Mention of trade names or
commercial products does not constitute endorsement or recommendation for use by the
US EPA. This report, contribution number AED #07-056, has been technically reviewed
by the US EPA’s Office of Research and Development, National Health and Environmental
Effects Research Laboratory, Atlantic Ecology Division, Narragansett, RI, and approved for
publication. Approval does not signify that the contents necessarily reflect the views and
policies of the Agency.
Literature Cited
Aravena, R.M., M.L. Evans, and J.A. Cherry. 1993. Stable isotopes of oxygen and nitrogen
in source identification of nitrate from septic systems. Ground Water 31:180–186.
Bannon, R.O., and C.T Roman, C. 2008. Using stable isotopes to monitor anthropogenic
nitrogen inputs to estuaries. Ecololgical Applications 18:22–30.
Bertness, M.D., P. Ewanchuk, and B.R. Silliman. 2002. Anthropogenic modification of
New England salt marsh landscapes. Proceedings of the National Academy of Sciences
of the United States of America 99:1395–1398.
Bigelow, H.B., and W.C. Schroeder. 1953. Fishes of the Gulf of Maine. Fishery Bulletin 74.
Fishery Bulletin of the Fish and Wildlife Service, Volume 53. Washington, DC.
Bowen, J.L., K.D. Kroeger, G. Tomasky, W.J. Pabich, M.L. Cole, R.H. Carmichael, and I.
Valiela. 2007a. A review of land-sea coupling by groundwater discharge of nitrogen to
New England estuaries: Mechanisms and effects. Applied Geochemistry 22:175–191.
Bowen, J.L., J.M. Ramstack, S. Mazzilli, and I. Valiela. 2007b. NLOAD: An interactive,
web-based modeling tool for nitrogen management in estuaries. Ecological Applications
17:S17–S30.
Cole, M.L., I. Valiela, K.D. Kroeger, B. Fry, G.I. Tomasky, J. Cebrian, C. Wigand, R. McKinney,
S. Grady, and M.E.C. Silva. 2004. Assessment of the isotopic method to indicate
anthropogenic eutrophication in coastal lagoons. Journal of Environmental Quality
33:124–132.
Collins, G., J.N. Kremer, and I. Valiela. 2000. Assessing uncertainty in estimates of nitrogen
loading to estuaries for research, planning, and risk assessment. Environmental
Management 25:635–645.
Northeastern Naturalist
8
C. Wigand, H. Smith, C. Spears, B. Keith, R. McKinney, M. Chintala, and K. Raposa
2015 Vol. 22, No. 1
Deacutis, C.F. 2008. Evidence of ecological impacts from excess nutrients in upper Narragansett
Bay. Pp. 349–382, In A. Desbonett, B. A. Costa-Pierce (Eds.). Science for
Ecosystem-based Management: Narragansett Bay in the 21st Century. Springer, New
York, NY.
Deegan, L.A., D.S. Johnson, R.S. Warren, B. Peterson, J.W. Fleeger, S. Fagherazzi, and W.
Wollheim. 2012. Coastal eutrophication as a driver of marsh loss. Nature 490:388– 392.
Diaz, R.J., and R. Rosenberg. 1995. Marine benthic hypoxia: A review of its ecological effects
and the behavioural responses of benthic macrofauna. Oceanography and Marine
Biology Annual Review 33:245–303.
Diaz, R.J., and R. Rosenberg. 2001. Overview of anthropogenically induced hypoxic effects
on Marine Benthic Fauna. Pp. 129–146, In N.N. Rabalais and R.E. Turner (Eds.).
Coastal Hypoxia: Consequences for Living Resources and Ecosystems. Coastal and
Estuarine Studies No. 58. American Geophysical Union, Washington, DC. 463 pp.
Freyer, H.D., and A.I.M. Aly. 1974. Nitrogen-15 variations in fertilizer nitrogen. Journal of
Environmental Quality 3:405–406.
Kinney, E.L., and I. Valiela. 2011. Nitrogen loading to Great South Bay: Land use, sources,
retention, and transport from land to bay. Journal of Coastal Research 27:672–686.
Kreitler, C.W., and D.C. Jones. 1975. Natural soil nitrate: The cause of the nitrate contamination
of ground water in Runnels county, Texas. Ground Water 13:53–61.
LaBrecque, E., C. Fritz, J. Tober, P.J. Behr, and I. Valiela. 1996. Abundance and age-specific
growth rates in relation to population densities of Fundulus heteroclitus in Waquoit Bay
estuaries subject to different nitrogen loadings. Biological Bulletin 191:319–320.
Latimer, J.S., and M. Charpentier. 2010. Nitrogen inputs to seventy-four southern New England
estuaries: Application of a watershed nitrogen loading model. Estuarine, Coastal,
and Shelf Science 89:125–136.
Macko, S.A., and N.E. Ostrom. 1994. Pollution studies using stable isotopes. Pp. 45–62,
In K. Lajtha and R.H. Michener (Eds.). Stable Isotopes in Ecology. Blackwell Scientific
Publications, London, UK.
McClelland, J.W., and I. Valiela. 1998. Linking nitrogen in estuarine producers to landderived
sources. Limnology and Oceanography 43:577–585.
McClelland, J.W., I. Valiela, and R.H. Michener. 1997. Nitrogen-stable isotope signatures
in estuarine food webs: A record of increasing urbanization in coastal watersheds. Limnolology
and Oceanography 42:930–937.
McKinney, R.A., W.G. Nelson, M.A. Charpentier, and C. Wigand. 2001. Ribbed Mussel
nitrogen isotope signatures reflect nitrogen sources in coastal salt marshes. Ecological
Applications 11:203–214.
Nixon, S.W. 1992. Quantifying the relationship between nitrogen input and the productivity
of marine ecosystems. Proceedings of the Advancement of Marine Technology Conference
5:57–83.
Nixon, S.W., S. Granger, B.A. Buckley, M. Lamont, and B. Rowell. 2004. A one-hundredand-
seventeen-year coastal water-temperature record from Woods Hole, Massachusetts.
Estuaries 27:397–404.
Nixon, S.W., B.A. Buckley, S.L. Granger, L.A. Harris, A.J. Oczkowski, R.W. Fulweiler,
and L.W. Cole. 2008. Nitrogen and phosphorus inputs to Narragansett Bay: Past, present,
and future. Pp. 101–175, In A. Desbonett and B.A. Costa-Pierce (Eds.). Science
for Ecosystem-based Management:Narragansett Bay in the 21st Century. Springer, New
York, NY.
Northeastern Naturalist Vol. 22, No. 1
C. Wigand, H. Smith, C. Spears, B. Keith, R. McKinney, M. Chintala, and K. Raposa
2015
9
Oczkowski A.J., S.W. Nixon, S.L. Granger, A-F. M. El-Sayed, and R. McKinney. 2009.
Anthropogenic enhancement of Egypt’s Mediterranean fishery. Proceedings of the US
National Academy of Sciences 106:1364–1367.
Pruell, R.J., B.K. Taplin, J.L. Lake, and S. Jayaraman. 2006. Nitrogen isotope ratios in
estuarine biota collected along a nutrient gradient in Narragansett Bay, Rhode Island,
USA. Marine Pollution Bulletin 52:612–620.
Silliman, B.R., and M.D. Bertness. 2004. Shoreline development drives invasion of Phragmites
australis and the loss of plant diversity on New England salt marshes. Conservation
Biology 18:1424–1434.
Tober, J.D., M.P.A Griffin, and I. Valiela. 2000. Growth and abundance of Fundulus heteroclitus
and Menidia menidia in estuaries of Waquoit Bay, Massachusetts, exposed to
different rates of nitrogen loading. Aquatic Ecology 34:299–306.
Valiela, I., G. Collins, J. Kremer, K. Lajtha, M. Geist, B. Seely, J. Brawley, and C.H. Sham.
1997. Nitrogen loading from coastal watersheds to receiving estuaries: New method and
application. Ecological Applications 7:358–380.
Valiela, I., M. Geist, J. McClelland, and G. Tomasky. 2000. Nitrogen loading from watersheds
to estuaries: Verification of the Waquoit Bay nitrogen loading model. Biogeochemistry
49:277–293.
Wigand, C., R. Comeleo, R. McKinney, G. Thursby, M. Chintala, and M. Charpentier. 2001.
Outline of a new approach to evaluate ecological integrity of salt marshes. Human and
Ecological Risk Assessment 7:1541–1554.
Wigand, C., R. McKinney, M. Chintala, M. Charpentier, and G. Thursby. 2003. Relationships
of nitrogen loadings, residential development, and physical characteristics with
plant structure in New England salt marshes. Estuaries 26:1494–1504.
Wigand, C., C.T. Roman, E.W. Davey, M.H. Stolt, R.L. Johnson, A. Hanson, E.B. Watson,
S.B. Moran, D.R. Cahoon, J.C. Lynch, and P. Rafferty. 2014. Below the disappearing
marshes of an urban estuary: Historic nitrogen trends and soil structure. Ecological Applications
24:633–649.