Physicochemical Controls on Spatiotemporal Distribution
and Benthic Mat Severity of Didymosphenia geminata in Pine Creek, an Unregulated Watershed in Northern
Pennsylvania
Matthew K. Shank
Northeastern Naturalist, Volume 26, Issue 2 (2019): 420–445
Full-text pdf (Accessible only to subscribers. To subscribe click here.)
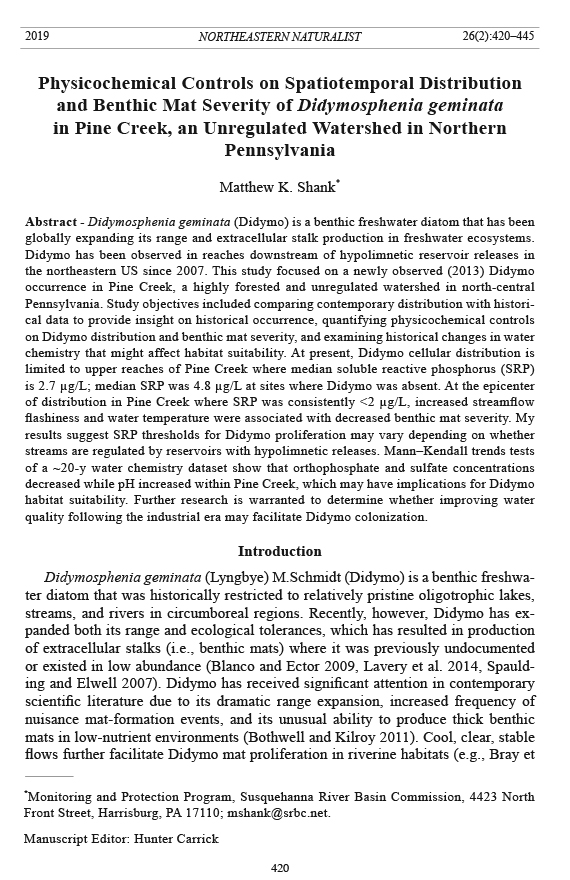
Access Journal Content
Open access browsing of table of contents and abstract pages. Full text pdfs available for download for subscribers.
Current Issue: Vol. 30 (3)
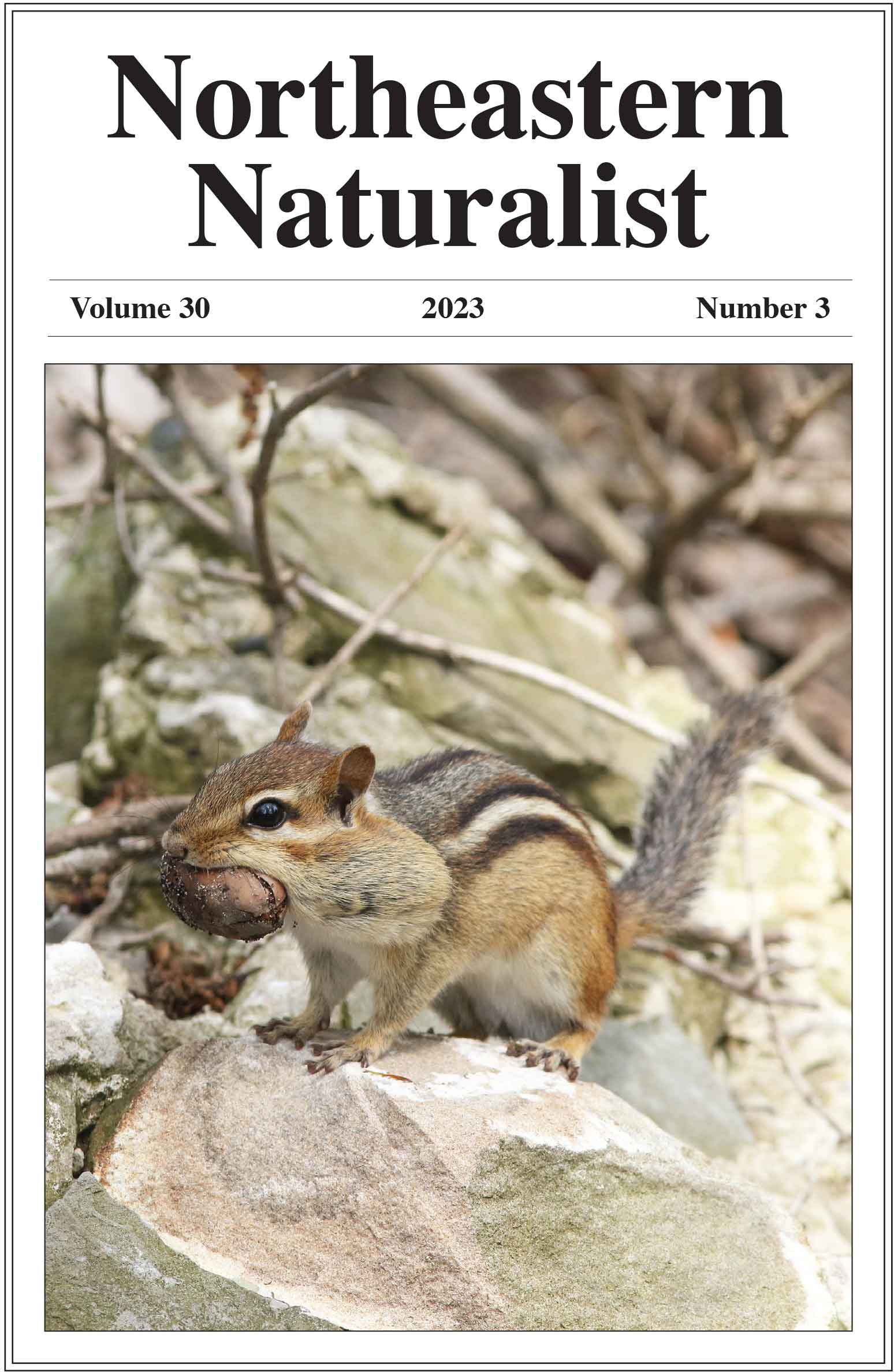
Check out NENA's latest Monograph:
Monograph 22
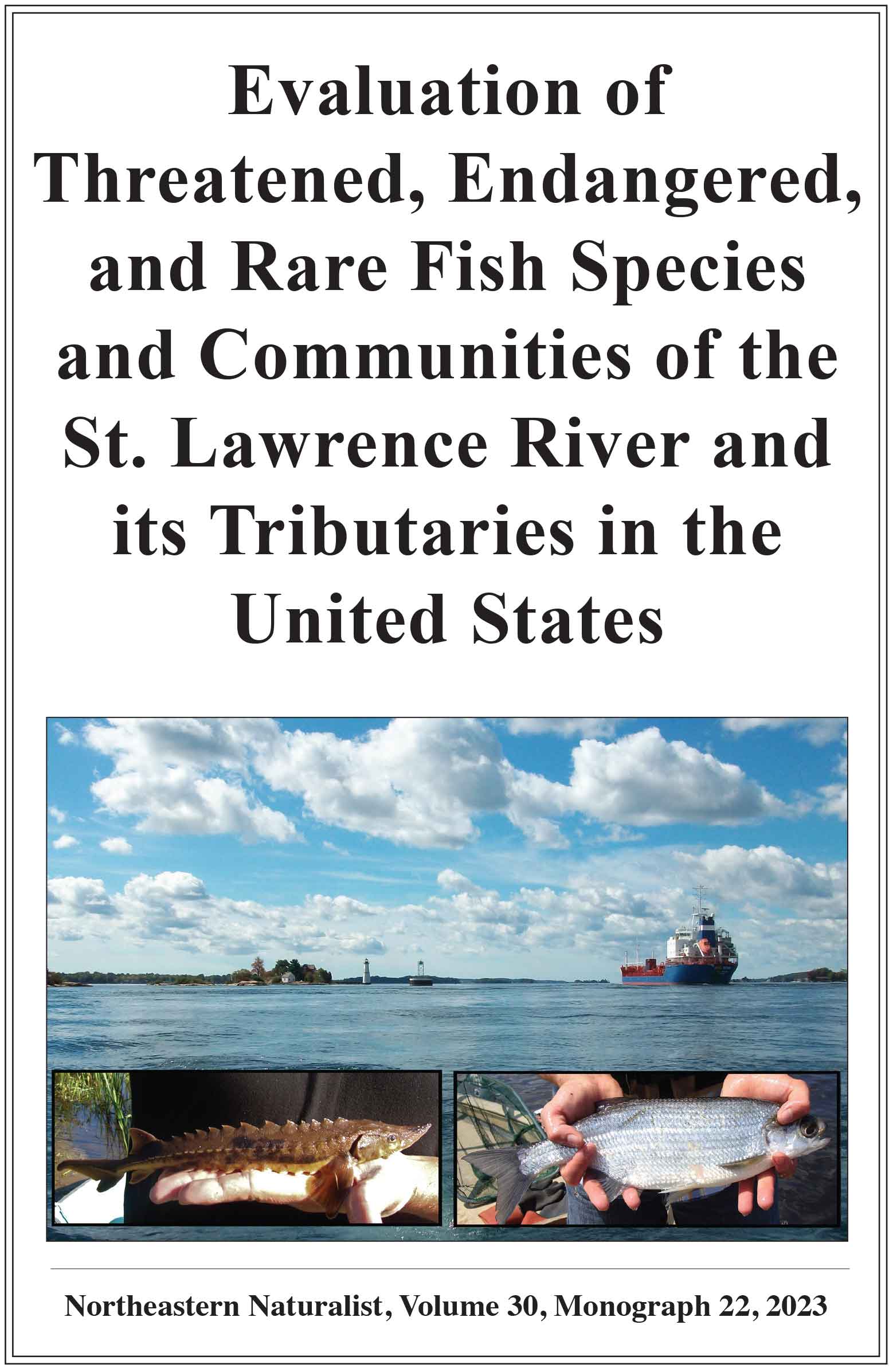
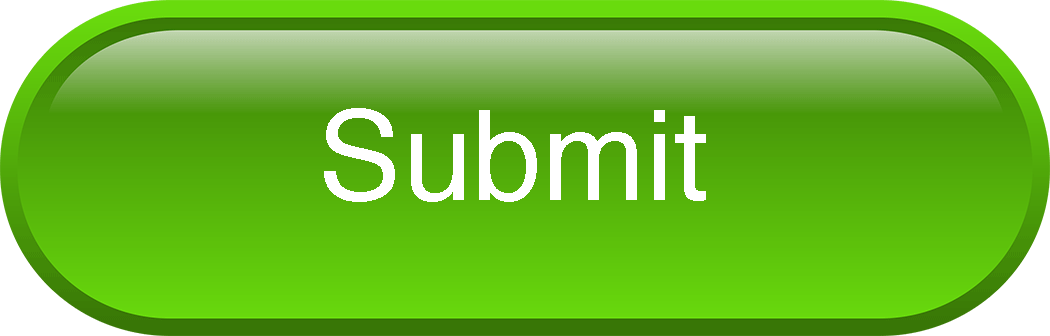
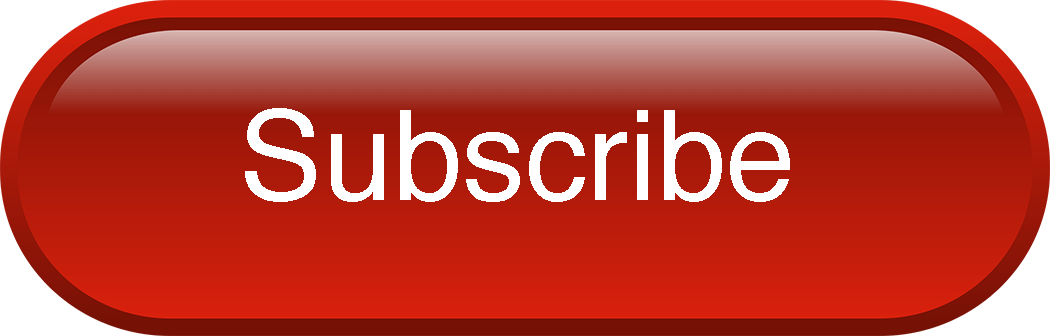
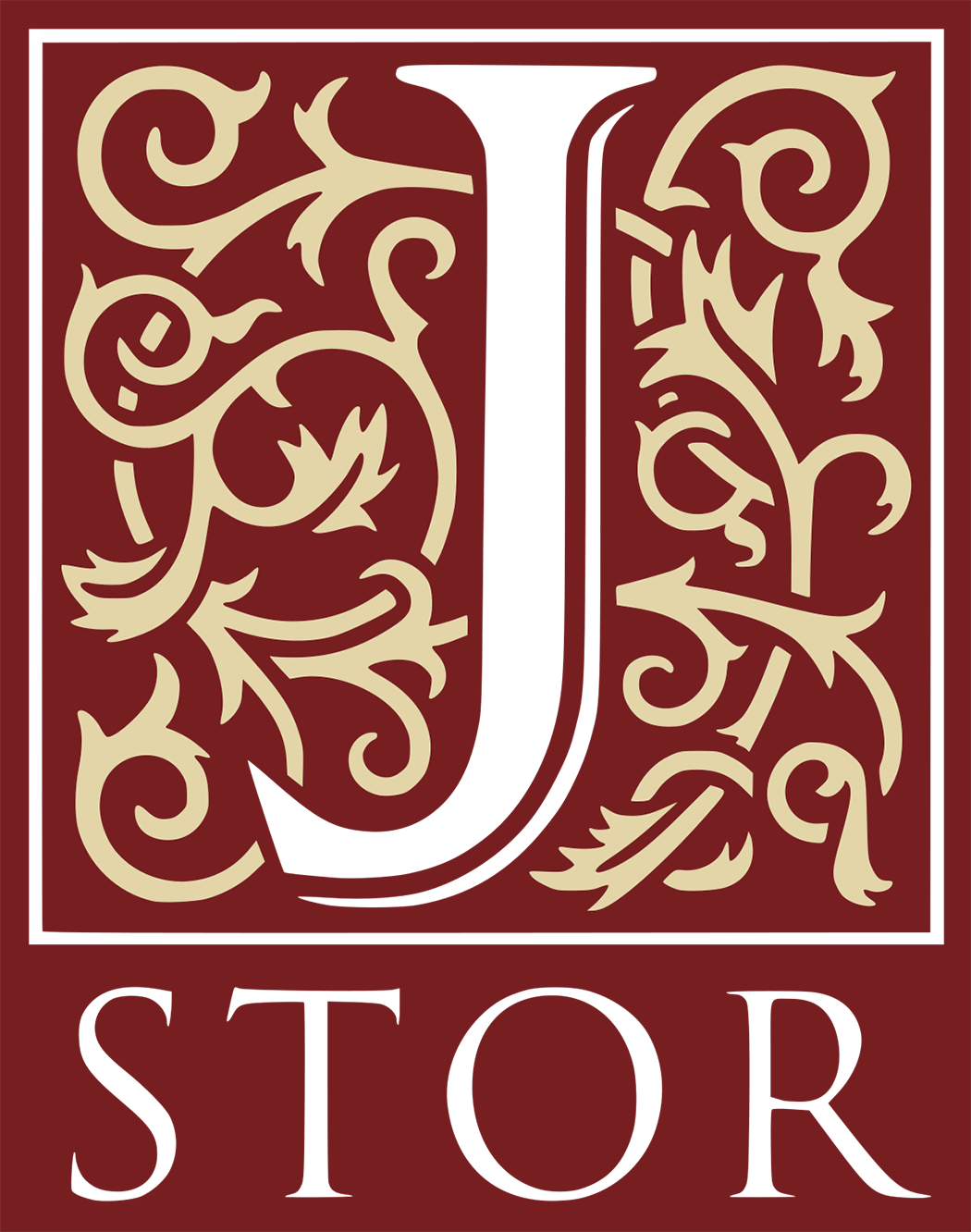
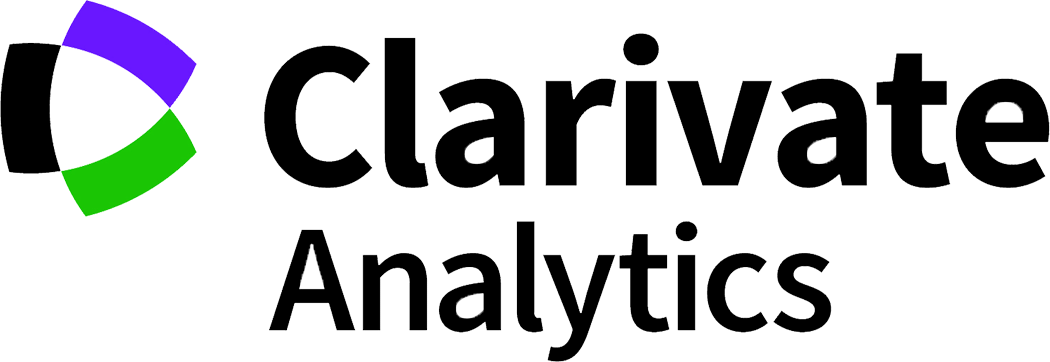
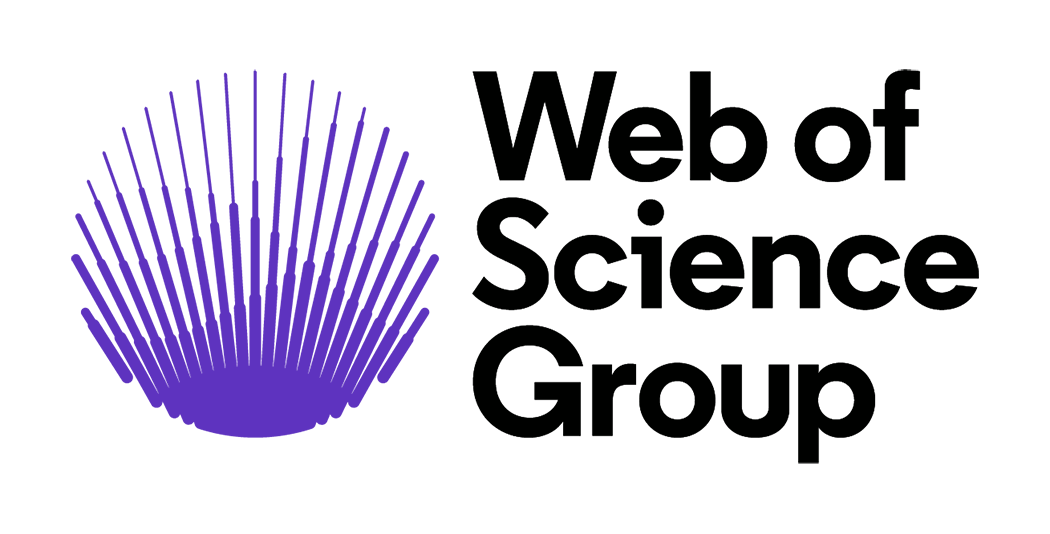
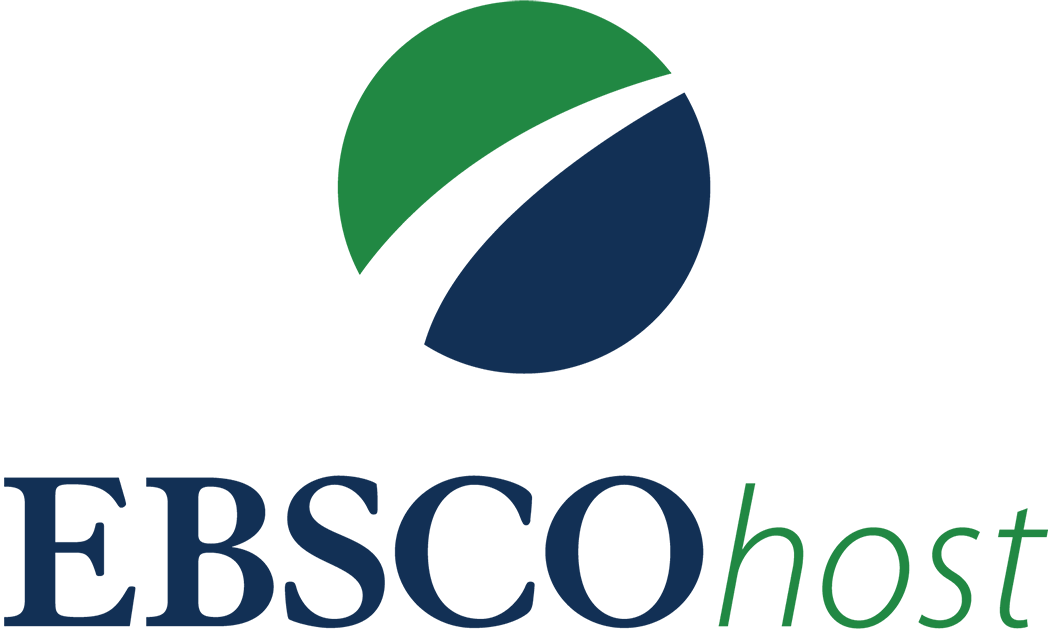
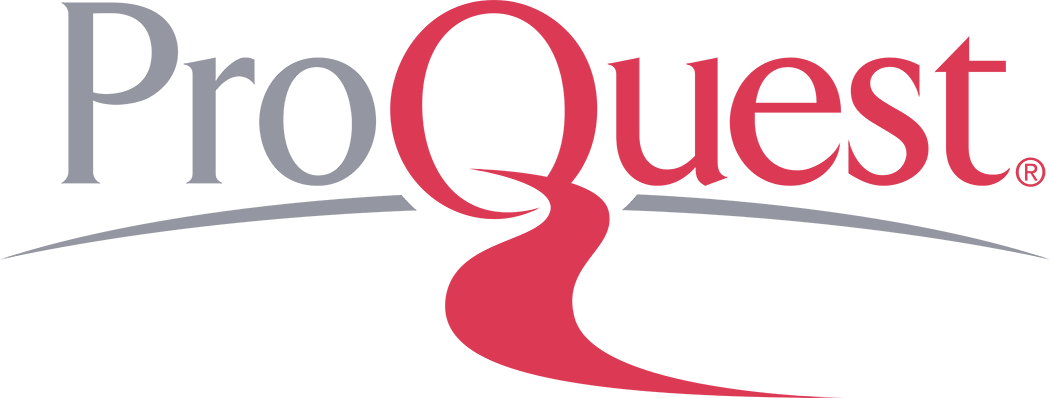
Northeastern Naturalist
420
M.K. Shank
22001199 NORTHEASTERN NATURALIST 2V6(o2l). :2462,0 N–4o4. 52
Physicochemical Controls on Spatiotemporal Distribution
and Benthic Mat Severity of Didymosphenia geminata
in Pine Creek, an Unregulated Watershed in Northern
Pennsylvania
Matthew K. Shank*
Abstract - Didymosphenia geminata (Didymo) is a benthic freshwater diatom that has been
globally expanding its range and extracellular stalk production in freshwater ecosystems.
Didymo has been observed in reaches downstream of hypolimnetic reservoir releases in
the northeastern US since 2007. This study focused on a newly observed (2013) Didymo
occurrence in Pine Creek, a highly forested and unregulated watershed in north-central
Pennsylvania. Study objectives included comparing contemporary distribution with historical
data to provide insight on historical occurrence, quantifying physicochemical controls
on Didymo distribution and benthic mat severity, and examining historical changes in water
chemistry that might affect habitat suitability. At present, Didymo cellular distribution is
limited to upper reaches of Pine Creek where median soluble reactive phosphorus (SRP)
is 2.7 μg/L; median SRP was 4.8 μg/L at sites where Didymo was absent. At the epicenter
of distribution in Pine Creek where SRP was consistently less than 2 μg/L, increased streamflow
flashiness and water temperature were associated with decreased benthic mat severity. My
results suggest SRP thresholds for Didymo proliferation may vary depending on whether
streams are regulated by reservoirs with hypolimnetic releases. Mann–Kendall trends tests
of a ~20-y water chemistry dataset show that orthophosphate and sulfate concentrations
decreased while pH increased within Pine Creek, which may have implications for Didymo
habitat suitability. Further research is warranted to determine whether improving water
quality following the industrial era may facilitate Didymo colonization.
Introduction
Didymosphenia geminata (Lyngbye) M.Schmidt (Didymo) is a benthic freshwater
diatom that was historically restricted to relatively pristine oligotrophic lakes,
streams, and rivers in circumboreal regions. Recently, however, Didymo has expanded
both its range and ecological tolerances, which has resulted in production
of extracellular stalks (i.e., benthic mats) where it was previously undocumented
or existed in low abundance (Blanco and Ector 2009, Lavery et al. 2014, Spaulding
and Elwell 2007). Didymo has received significant attention in contemporary
scientific literature due to its dramatic range expansion, increased frequency of
nuisance mat-formation events, and its unusual ability to produce thick benthic
mats in low-nutrient environments (Bothwell and Kilroy 2011). Cool, clear, stable
flows further facilitate Didymo mat proliferation in riverine habitats (e.g., Bray et
*Monitoring and Protection Program, Susquehanna River Basin Commission, 4423 North
Front Street, Harrisburg, PA 17110; mshank@srbc.net.
Manuscript Editor: Hunter Carrick
Northeastern Naturalist Vol. 26, No. 2
M.K. Shank
2019
421
al. 2016, Cullis et al. 2012, Kirkwood et al. 2009), which, in addition to degrading
the experience of recreationists, has the potential to alter physical and biological
conditions in aquatic ecosystems and negatively impact local economies (Beville
et al. 2012, Spaulding and Elwell 2007, Whitton et al. 2009).
Didymo appears to have been introduced to New Zealand (Kilroy and Novis
2018, Kilroy and Unwin 2011), but other locations closer to the historic range of
this species (i.e., the northeastern US) lack conclusive data concerning the origin
of this organism. Didymo has existed on the North American continent for at least
11,000 y, as evidenced by a fossilized specimen collected in British Columbia
(Letham et al. 2016). Didymo has been present and stable in abundance in southern
Alaska for ~800 y (Pite et al. 2009). Records from the northeastern US include
subfossil evidence of Didymo presence on Long Island, NY (Lohman 1939),
from the Delaware River near Philadelphia, PA (Boyer 1916, 1927), and from
an unknown watershed in Virginia in the late 20th century (Patrick and Reimer
1975). However, Didymo mats were not visually observed in surface waters in the
northeastern US until around 2007, and it has since colonized reaches influenced
by hypolimnetic reservoir releases in the Delaware River, Youghiogheny River,
Savage River, and Gunpowder Falls, among others (Keller et al. 2017, Klauda
and Hanna 2016, Shank et al. 2016). For a time, the presence of Didymo seemed
limited to settings with hydrologic and thermal regulation provided by reservoirs.
Then, in June 2013, Didymo was detected in the middle portion of the mainstem
of Pine Creek in Lycoming County near Hamilton Bottom, PA. This initial observation
consisted of Didymo cells collected using a plankton-tow net in the
water column. In October 2013, Didymo was found attached to the substrate 80
km further upstream on West Branch Pine Creek in Potter County near Galeton,
PA (Shank et al. 2016). Pine Creek is an unregulated watershed that is largely
forested and considered recreationally and ecologically valuable. The absence of
dams in the watershed, and the resulting lack of hydrologic and thermal controls,
makes the Didymo colony present in Pine Creek unique in the context of Didymo
proliferation in the northeastern US.
Didymo is capable of producing nuisance benthic mats up to 20 cm thick characterized
by exorbitant growth of extracellular stalks, which may comprise up to 90%
of Didymo biomass (Whitton et al. 2009). Recent research has led to key insights
into physicochemical variables that govern abundance and distribution of Didymo,
including streamflow regulation, geomorphic characteristics, watershed disturbance,
soil type, water chemistry, geology, and turbidity. A full synthesis of the
associations of Didymo with these variables is beyond the scope of this manuscript,
but has been completed elsewhere (i.e., Blanco and Ector 2009, Bray et al. 2016,
Cullis et al. 2012, Whitton et al. 2009). Due to the regional nature of this work, I focus
on a limited number of physicochemical variables. Foremost is soluble reactive
phosphorus (SRP), which at concentrations less than 2 μg/L results in decreased Didymo
cell division but stimulates stalk production (Bothwell and Kilroy 2011, Bothwell et
al. 2014). James et al. (2015) demonstrated that adding phosphorus (P) significantly
Northeastern Naturalist
422
M.K. Shank
2019 Vol. 26, No. 2
decreased Didymo mat biomass in Rapid Creek, SD. The combination of low SRP
and cool, clear, stable flows has been shown to increase Didymo mat severity (e.g.,
Bray et al. 2016; Cullis et al. 2012; Kilroy and Bothwell 2012, 2014; Kirkwood et
al. 2009). Although mechanisms for Didymo mat development have been identified,
thresholds other than less than 2 μg/L SRP have not been thoroughly quantified and
may be regionally specific (Cullis et al. 2012, Kunza et al. 2018). Further, the role
of changing environmental conditions has been posited as a possible reason for
global Didymo proliferation. Deposition of nitrogen (N) from burning fossil fuels,
which in concert with earlier growing seasons and hydrologic shifts due to climate
change, could be causing P limitation that favors Didymo over broad scales (Bothwell
et al. 2014, Taylor and Bothwell 2014). The improvement of water quality in
the northeastern US following the end of industrial and resource extraction eras,
coupled with air pollution regulations that reduce stream acidification (Stets et al.
2012, Stoddard et. al 1999) could also be factors in creating suitable habitat for
Didymo, which has gone unstudied to date.
In this study, I focused on 4 objectives that are central to the ecological understanding
of Didymo on a regional scale. The first objective was to compare
historical algal data in Pine Creek with contemporary Didymo cellular distribution
data to provide insight to the ongoing debate related to the native or non-native
status of Didymo. Second, I analyzed SRP concentrations at sites with and without
Didymo cellular presence to determine if the less than 2-ug/L threshold for mat proliferation
is appropriate in the northeastern US. Third, I developed a novel approach
using continuous monitoring of stream temperature, turbidity, and flow at the epicenter
of Didymo in Pine Creek to quantify the associations between the measured
variables and Didymo mat severity. Lastly, I used Mann–Kendall trend tests to analyze
a ~20-y water chemistry, discharge, and air-temperature dataset from a sentinel
monitoring location within Pine Creek to determine if environmental conditions are
changing, which could have implications for Didymo colonization.
Field Site Description
The Pine Creek watershed, which has a total drainage area of 2538 km2 and
is 89% forested (USGS 2016), is located within north-central Pennsylvania (PA)
in Potter, Tioga, and Lycoming counties (Fig. 1). Today, high-quality headwater
streams supporting Salvelinus fontinalis (Mitchill) (Brook Trout) and Salmo trutta
L. (Brown Trout) contribute to Pine Creek, which flows through the “Pennsylvania
Grand Canyon” and is classified as a PA Scenic River. A history of industry
and resource extraction during the colonial period caused large-scale degradation
of aquatic resources. Beginning around 1800, tanneries and coal mines increased
the concentrations of metals and acidity in Pine Creek, while logging reduced the
prolific Pinus strobus L. (White Pine) and Tsuga canadensis (L.) Carrière (Eastern
Hemlock) forests to bare ground throughout much of the watershed by the early
1900s (Detar and Kristine 2012). The health of Pine Creek has steadily increased
during the post-industrial period, but the lasting effects of the millions of acreNortheastern
Naturalist Vol. 26, No. 2
M.K. Shank
2019
423
feet of logs transported to lumber mills downstream via Pine Creek is still largely
responsible for the geomorphology of Pine Creek and many of its tributaries today
(Detar and Kristine 2012).
Figure 1. Mean soluble reactive phosphorus (SRP) concentrations in relation to D. geminata
presence and cell density at monitoring locations throughout the Pine Creek watershed. The
monitoring location labeled “B” is collocated with the West Branch Pine Creek (WPIN)
intensive monitoring site.
Northeastern Naturalist
424
M.K. Shank
2019 Vol. 26, No. 2
Methods
Didymo spatial distribution in relation to soluble reactive phosphorus
I completed a systematic search for periphyton/algal assemblage records in the
Pine Creek watershed to serve as evidence of Didymo cell presence or absence
prior to the first known observation of cells in 2013. I compile d data from floristic
surveys (Potapova 2010) and diatom monitoring efforts of agencies (Susquehanna
River Basin Commission [SRBC], Pennsylvania Department of Environmental
Protection [PADEP], US Environmental Protection Agency [USEPA]) and academic
institutions (Academy of Natural Sciences PA [ANSP]). These data were
collected using various techniques including natural-substrate scrapes, tow-net
deployment in the water column, and artificial tiles.
I responded to the appearance of Didymo in Pine Creek with intensive surveys
to determine contemporary distribution. I sampled all major tributaries in the
watershed (>3rd Strahler stream order) using environmental DNA (eDNA) data
collection that employed a plankton drift net with 35-μm mesh and fitted with a
250-μm-mesh pre-filter. The eDNA samples were analyzed using a quantitative
real-time polymerase chain reaction procedure (Cary et al. 2014). Specific methods
and results of the regional eDNA survey, which included 32 samples from 16 sites
in Pine Creek during 2014–2015 are detailed by Keller et al. (2017). In addition
to eDNA surveys, I collected 23 periphyton “rock scrape” samples from 15 sites
within the watershed. I collected all rock scrape samples using modified Rapid
Bioassessment Protocols methods (Barbour et al. 1999), where 11 pieces of natural
substrate (cobbles) were removed uniformly from the entire width of the stream at
each site. I used a soft brush to disturb periphyton within a 12-cm2 delimiter from
each cobble, rinsed the material into a composite sample bottle, and recorded the
corresponding sample volume. I used formaldehyde to preserve a 50-mL subsample
of the composite sample. In the laboratory, M. Potapova created permanent slides
and examined them using microscopy to determine presence or absence of Didymo
cells following ANSP (2002) protocol. I collected 10 of the rock-scrape samples
from 5 sites along a 39-km longitudinal section of Pine Creek in spring and fall
2015 to examine the spatial and temporal dynamics of Didymo cell density. Specifically,
300–500 valve counts were conducted to determine diatom taxa density.
When Didymo was present but relative abundance was low, stratified counts were
also conducted to determine Didymo density with increased accuracy.
I collected water samples at 12 sites within the Pine Creek watershed (Fig. 1).
I sampled each site 7–20 times during 2014–2015, for a total of 106 samples. I
measured SRP and total dissovled phosphorus (TDP) concentrations (minimum
detection limit = 1.1 μg/L and 7.8 μg/L, respectively) due to the association with
Didymo mat formation (Bothwell and Kilroy 2011). I performed a 2-sample t-test
in R to test for significant differences (α = 0.05) in SRP concentration at sites based
on presence or absence of Didymo cells (R Core Team 2016).
Didymo mat severity in relation to physicochemical variables
I established an intensive monitoring site on the West Branch of Pine Creek
(WPIN), which is the epicenter of the Didymo colony in the watershed (Fig. 1).
Northeastern Naturalist Vol. 26, No. 2
M.K. Shank
2019
425
The high-resolution physicochemical data collected at this location were intended
to elucidate relationships between physicochemical parameters and Didymo mat severity.
I deployed a YSI 6600 data sonde (YSI, Inc., Yellow Springs, OH) to collect
pH, specific conductance, water temperature, dissolved oxygen, and turbidity data
at 15-min intervals (SRBC 2017). In addition, I characterized underwater photosynthetically
active radiation (PAR) with a sensor (single LI-COR 2-pi, wiped; Licor,
Lincoln, NE) fastened to the substrate using a rebar stake, and integrated into the
existing data sonde. I made 16 Didymo benthic mat severity measurements (standing
crop index; SCI) between April 2014 and December 2016 at WPIN. I calculated
mean SCI by averaging percent coverage * mat thickness (mm) from 11 pieces of
substrate that were removed from the entire width of the stream. SCI is highly correlated
with periphyton ash-free dry mass (AFDM), and an SCI threshold of 220
(equivalent to 35 g/m2 AFDM) approximates a threshold for nuisance periphyton
(Kilroy and Bothwell 2012). SCI is considered a surrogate for cell density, but best
represents mat severity due to the large proportion of extracellular material that can
be present even as cell densities remain low. For instance, Didymo cell densities
did not exceed 3% of the entire diatom community in several studies, even when
extracellular mats were a dominant feature on the substrate (George and Baldigo
2015, Gillis and Lavoie 2014, Spaulding and Elwell 2007). SCI is a widely used
standardized metric that allows direct comparisons on local to global scales (Gillis
et al. 2018, Kilroy and Bothwell 2012).
Onsite streamflow monitoring at WPIN was not available; however, we used
a hybrid of streamflow-record extension (Hirsch 1982) and drainage-area ratio
(Hirsch 1979) methods to model average daily streamflow (ADF) at WPIN.
Specifically, 35 discrete discharge measurements were collected at WPIN since
2010 that spanned a large range of hydrologic conditions. I used these discharge
measurements to develop a regression equation with the most spatially correlated,
unregulated US Geological Survey (USGS) stream gage for comparison of conditions
at WPIN, which was determined to be gage 01544500 on Kettle Creek at
Cross Fork, PA. The relationship of log10-transformed observed discharge measurements
at WPIN and ADF at Kettle Creek was significant (P < 0.001; R² = 82%).
To avoid the potential biases associated with extrapolating high flow outside of
the range where measurements were used to derive the regression equation, I used
the drainage-area ratio method (Hirsch 1979) to determine all ADF values greater
than the maximum observed discharge. Once the time-series hydrograph had been
generated, I calculated flow-variability metrics, including the flow coefficient of
variation and Richards–Baker flashiness index. The latter measures oscillations
relative to the cumulative streamflow to indicate the flashiness of streamflow during
the time span (Equation 2 in Baker et al. 2004). I collected channel geometry
measurements at the site using modified USGS National Water Quality Assessment
Program protocols (Fitzpatrick et al. 1998). I modeled average channel-bed shear
stress for a streamflow event that would result in mobility of coarse gravel (32-mm
particle size) and defined the streamflow value that corresponded to this level of
shear stress.
Northeastern Naturalist
426
M.K. Shank
2019 Vol. 26, No. 2
Water chemistry sampling at WPIN resulted in 19 observations of SRP between
April 2014 and January 2016. I used these discrete measurements to predict
continuous concentrations. Although total P concentrations in streams are known
to correlate with streamflow (Ruzycki et al. 2014), it is less clear whether SRP
concentrations are likewise correlated. Streamflow was significantly positively
correlated with SRP, which and had the strongest relationship with streamflow of
all continuous variables collected (Pearson r = 0.648, P = 0.007). I employed the
composite method (function loadComp) in the loadflex package (Appling et. al
2015, Lorenz et al. 2015) in the R software environment (R Core Team 2016) to
estimate daily SRP concentrations based on empirical observations and streamflow.
Once I completed data collection and generated time-series datasets, I summarized
onditions prior to each Didymo mat severity sample for temporal periods
of 7 d, 15 d, 30 d, 60 d, 90 d, and 120 d. These antecedent conditions summarized
flow variability metrics and number of days where flow exceeded critical shear
stress values. I also calculated mean water temperature and turbidity. I selected
the antecedent period of each variable that explained the greatest amount of variation
(adjusted R2) to serve as predictor variables in a series of linear regression
models to describe variation in mat severity at WPIN. Subsequently, I employed
the information theoretic approach (Burnham and Anderson 2002) to determine if
streamflow, stream temperature, turbidity, or all variables best explained variation
in mat severity. These candidate models reflected a priori hypotheses that cool,
clear, stable streamflows facilitate Didymo mat proliferation (e.g., Bray et al. 2016,
Cullis et al. 2012, Kirkwood et al. 2009). I chose Akiake’s information criterion
corrected for small sample size (AICc) to determine the level of support for the 4
competing models. The model with the lowest AICc value had the most support,
while competing models had a Δi (AICi - AICmin) ~ 2. Mat severity (SCI) was
square-root transformed in all models to ensure that homoscedasticity was attained,
residuals were approximately normally distributed, and individual observations did
not have undue influence on the relationship. All analyses were performed in R (R
Core Team 2016).
Changes in water chemistry
I obtained water chemistry data from a long-term monitoring site on the mainstem
of Pine Creek near Waterville, PA (PADEP Water Quality Network site 410),
where data had been collected approximately bimonthly from 1998 to 2016. This
site is located on Pine Creek 62 km downstream from the nearest location where
Didymo has been observed on the substrate (Fig. 1); however, this site represents
a sentinel monitoring station where conditions are representative of the entire
drainage and where the longest-term dataset is available. I retrieved and cleaned
data from STORET (USEPA 2017), and selected parameters for analysis that
have been shown to correlate with Didymo cell presence and/or mat proliferation.
Total aluminum, iron, N, orthophosphate, P, sulfate, and pH contained relatively
complete records, as did other surrogates for water quality, including total dissolved
solids and specific conductance. Spearman tests indicated that all of these
Northeastern Naturalist Vol. 26, No. 2
M.K. Shank
2019
427
parameters were significantly correlated with average daily stream flow (α =
0.05), which I obtained from the USGS streamflow gage 01549700 on Pine Creek
in Waterville, PA (Fig. 1). Flow-adjusted Mann-Kendall trend tests were completed
on these parameters to determine direction and significance of monotonic
trends during the study period. Also, I aggregated average daily streamflow and
air temperature for Williamsport, PA (37 km away from WQN 410; Pennsylvania
State Climatologist 2017) to monthly values and examined that data using correlated
seasonally adjusted Mann–Kendall tests. I considered trends significant
at α = 0.05; all analyses of trends were completed using the trend package in R
(Pohlert 2016, R Core Team 2016).
Results
Didymo spatial distribution in relation to soluble reactive phosphorus
I compiled and/or examined a total of 112 algal records that pre-dated the first observation
of Didymo in Pine Creek, which were collected between September 2008
and October 2012. None of these samples contained any evidence of the presence
of Didymo cells or mats in the Pine Creek watershed prior to June 2013, including 3
archived samples from June and July 2012 that were within the reach where Didymo
was observed attached to the substrate in 2013 (Fig. 2a). The intensive eDNA and
microscopy monitoring effort enacted in response to the first visual observation
of Didymo resulted in the collection of an additional 88 samples from June 2013
Figure 2. Didymosphenia geminata cellular presence and absence (a) before and (b) after
the first observation in the Pine Creek watershed in June 2013.
Northeastern Naturalist
428
M.K. Shank
2019 Vol. 26, No. 2
to December 2016. This effort revealed that Didymo cells were present beginning
in West Branch Pine Creek and, from there, directly downstream throughout Pine
Creek. There was no evidence that Didymo cells were present in any of the tributaries
where sampling was conducted. Didymo cells were detected in the water column up
to 101 km downstream from the upstream-most observation, while cells were present
on the substrate for 39 km (Fig. 2b). I visually observed Didymo mats on the substrate
for ~6 km downstream of the most upstream colony at WPIN. Notably, Didymo cells
were not present in 7 samples (2 eDNA and 5 microscopy) that were collected at the
most upstream site on West Branch Pine Creek between November 2013 and May
2015. Didymo mats were then observed attached to the substrate at this site in November
2015, indicating possible upstream range expansion.
Didymo mat severity is highest in upstream reaches, including West Branch Pine
Creek and upper Pine Creek. This finding is consistent with Didymo cell density,
which peaks near the mouth of West Branch Pine Creek and then falls precipitously
as tributaries with higher SRP concentrations and waste-water treatment discharges
enter Pine Creek (Fig. 1). SRP had nearly tripled (from 2.0 ± 0.9 to 5.7 ± 2.1 μg/L;
mean ± 1 standard deviation) 39 km downstream from the site with the highest
Didymo cell density, while cell density decreased from 3130 ± 3730 to 6.6 ± 9.4
cells/cm2 (Figs. 1, 3a).
Similarly, the presence or absence of Didymo cells appears to be associated
with the concentration of SRP in tributaries throughout the Pine Creek watershed.
Didymo has colonized West Branch Pine Creek, which contains the lowest
SRP concentration of all tributaries in the watershed. All other tributaries, where
Didymo was absent, have mean SRP concentrations >4 μg/L except for Little Pine
Creek (Fig. 1). SRP is significantly lower at sites where Didymo was present (3.8
± 4.7 μg/L, n = 46) compared with sites where Didymo was absent (6.6 ± 6.1 μg/L,
n = 60) (2-sample t-test, P = 0.004). The SRP means for both groups were inflated
due to high concentrations in a tributary site with no Didymo (Marsh Creek) and at
a site on Pine Creek containing Didymo directly downstream of a waste-water treatment
discharge during summer low-flow conditions (Fig. 3b). Therefore, median
values, which were 2.7 μg/L and 4.8 μg/L at sites where Didymo cells were absent
and present, respectively, may be more representative of the association between
SRP and Didymo presence.
Didymo mat severity in relation to physicochemical variables
At the epicenter of Didymo colonization (WPIN), where I conducted intensive
data collection, West Branch Pine Creek is a 4th order stream with a highly forested
watershed and slightly acidic pH, which represents a typical montane stream in
north-central Pennsylvania. There is a small (17.4 ha) impoundment on an upstream
tributary (Lyman Run; Fig. 1) that impounds ~20% of the watershed. This impoundment
is a simple spill-over design that does not have flood-storage capability and
thus does not result in the dampening of high flows during runoff events or in lowflow
augmentation. SRP at WPIN is among the lowest in the region (1.94 ± 0.95
μg/L), with the highest values (max = 4.0 μg/L) observed in the summer months
Northeastern Naturalist Vol. 26, No. 2
M.K. Shank
2019
429
during low-flow conditions. SRP modeling revealed consistently low values, with
concentrations less than 2.0 μg/L and less than 4.0 μg/L during 50% and 96% of days, respectively
(Fig. 4). Other water chemistry parameters are well within ranges supporting Didymo
presence elsewhere (Table 1; Whitton et al. 2009).
A total of 16 observations of Didymo mat severity (SCI) were made at WPIN
between April 2014 and December 2016. The SCI values varied from 0.0 to 283.5,
with only 1 observation above the nuisance periphyton threshold of 220 (Kilroy and
Bothwell 2012). Streamflow showed typical patterns of high seasonal flow events in
Figure 3. (a) Scatter plot of mean soluble reactive phosphorus (SRP) concentration and
Didymosphenia geminata (Didymo) cell density at 5 sites on Pine Creek and 7 tributary
sites. (b) Box plots of SRP concentration at sites where Didymo cells were present or absent.
Black triangles indicates mean values, bold lines indicate medians, lower and upper extent
of boxes indicate 1st and 3rd quartiles, respectively. Dashed line indicates SRP detection limit
of 1.1 μg/L. Continuous axes are square-root transformed to allow for clear visualization of
SRP and cell density at low values.
Northeastern Naturalist
430
M.K. Shank
2019 Vol. 26, No. 2
Figure 4. Frequency distribution of soluble reactive phosphorus concentrations at West
Branch Pine Creek (WPIN) where Didymosphenia geminata mat formation was intensively
monitored. Shaded polygons indicate 25th, 50th, and 75th percentiles.
Table 1. Physical and chemical characteristics of a continuously monitored site on West Branch Pine
Creek (WPIN). Values are shown as mean ± 1 standard deviation.
Variable Value Units
Didymosphenia geminata standing crop index 83.7 ± 87.5 -
Physical
Drainage size 182.1 km2
Proportion impounded 20.8 %
Forested area 97.0 %
Slope 0.6 %
Average daily streamflow 3.3 ± 3.4 m3/s
Substrate size 159.7 ± 171.7 mm
Width 14.0 ± 2.8 m
Annual water temperature 8.6 ± 6.5 °C
Summer water temperature 17.5 ± 2.8 °C
Dissolved oxygen 12.6 ± 2.1 mg/L
Chemical
Soluble reactive phosphorus 1.94 ± 0.95 μg/L
Total dissolved phosphorus* 7.85 ± 0.20 μg/L
Total phosphorus 18.62 ± 19.06 μg/L
Nitrate 0.50 ± 0.16 mg/L
Sulfate 6.36 ± 0.78 mg/L
Calcium 4.64 ± 0.59 mg/L
Total organic carbon 1.15 ± 0.82 mg/L
Turbidity 3.62 ± 5.45 NTU
Specific conductance 0.043 ± 0.005 ms/cm
pH 6.82 ± 0.35 SU
*Most observations are below the detection limit of 7.80 μg/l.
Northeastern Naturalist Vol. 26, No. 2
M.K. Shank
2019
431
winter and spring, with prolonged low flows in summer and fall in 2014 and 2016.
Flows were flashier throughout the summer of 2015, with numerous precipitation
runoff events resulting in a flashier hydrograph. Average daily water temperature
showed a seasonal pattern, with temperatures near 0 °C during winter months, and
occasionally exceeding 20 °C in the summer months (Fig. 5D). Stream turbidity
was low throughout the study period, with periodic peaks coinciding with high
intensity precipitation events that resulted in elevated stream flows (Fig. 5).
Figure 5. Plot of (a) Didymosphenia geminata observed standing crop index (SCI) values.
(b) average daily soluble reactive phosphorus, (c) streamflow, (d) water temperature, and
(e) stream turbidity, from January 2014 to December 2016 at the intensive monitoring site
on West Branch Pine Creek (WPIN).
Northeastern Naturalist
432
M.K. Shank
2019 Vol. 26, No. 2
Richards–Baker flashiness index, mean water temperature, and mean turbidity in
7-d, 30-d, and 15-d antecedent windows described 25.2%, 14.1%, and 8.9% of variation
in mat severity, respectively. These variables in their respective antecedent
windows had the highest adjusted R2 values when compared to other time periods
of each respective variable. ΔAICc values indicated that the streamflow flashiness
model received the most support, while the stream temperature model was considered
competing and there was limited support for the stream turbidity model and the
full model containing all variables (Table 2). As streamflow flashiness, temperature,
and turbidity increased, mat severity decreased (Fig. 6).
Changes in water chemistry
Water chemistry within Pine Creek has changed significantly since 1998. Orthophosphate
and sulfate show decreasing trends, while pH and specific conductance
have increased (Table 3, Fig. 7). I observed insignificant trends in the remainder of
the water chemistry parameters examined. Streamflow and air temperature showed
no overall trend, but air temperature showed significant increasing trends in the
months of July and October.
Discussion
Didymo spatial distribution in relation to soluble reactive phosphorus
The intensive data collection that followed the first observation of Didymo in
Pine Creek coupled with the historic data available in the watershed combine to
provide insight into which physicochemical variables influence the spatiotemporal
Figure 6. Relationship between Didymosphenia geminata mat severity and the 3 antecedent
variables representing a priori hypotheses: (a) Richards–Baker flashiness index, (b) mean
stream temperature, and (c) mean stream turbidity. Length of antecedent window is shown
in square brackets under x axis label.
Northeastern Naturalist Vol. 26, No. 2
M.K. Shank
2019
433
Table 2. Results of regression model-selection process reflecting a prior hypotheses on the effect of streamflow flashiness, temperature, and turbidity on
the square-root transformed standing crop index (SCI) of D. geminata at the intensive monitoring site on West Branch Pine Creek (WPIN). Length of
antecedent window is shown in square brackets in the parameter estimates columns. Parameter estimates ± standard error are shown.
Parameter estimates
Richards–Baker Mean water Mean turbidity
Intercept flashiness temp. (ºC) (NTU)
Model Type df P Multiple R2 Adjusted R2 AICc ΔAICc (sqrt SCI) index (7 days) (30 days) (15 days)
Streamflow 14 0.03 30.2% 25.2% 100.07 0.00 13.06 ± 2.63 -34.69 ± 14.11
Stream temperature 14 0.08 19.9% 14.1% 102.27 2.20 11.88 ± 2.78 -0.48 ± 0.26
Stream turbidity 14 0.14 15.0% 8.9% 103.22 3.15 8.21 ± 1.41 -0.017 ± 0.011
Full model 12 0.11 38.3% 22.9% 107.22 7.15 13.76 ± 2.91 -20.55 ± 18.98 -0.25 ± 0.31 -0.012 ± 0.011
Northeastern Naturalist
434
M.K. Shank
2019 Vol. 26, No. 2
Table 3. Results of Mann–Kendall trend tests for water chemistry variables, streamflow, and air temperature at the sentinel monitoring location on Pine
Creek (WQN 410). The date range, mean, standard deviation (SD), and number of ob servations (n) are included for each parameter.
Trend test type/ Parameter
Water chemistryparameter Covariate Z P Trend direction Date range mean ± SD n
Mann-Kendall Flow Adjusted
Aluminum Total (mg/L) Flow (m3/s) 1.275 0.202 Positive 10/13/98–9/19/16 0.28 ± 0.75 180
Iron total (mg/L) Flow (m3/s) 1.593 0.111 Positive 10/13/98–9/19/16 0.43 ± 1.20 180
Nitrogen total (mg/L) Flow (m3/s) -0.867 0.386 Negative 4/10/02–9/19/16 0.37 ± 0.25 157
Orthophosphate total (mg/L) Flow (m3/s) -2.810 0.005 Negative** 4/10/02–9/19/16 0.007 ± 0.005 158
Phosphorus total (mg/L) Flow (m3/s) -0.705 0.481 Negative 10/13/98–9/19/16 0.025 ± 0.037 133
pH Flow (m3/s) 4.827 0.000 Positive** 10/13/98–9/19/16 7.41 ± 0.35 181
Specific conductance (ms/cm) Flow (m3/s) 2.051 0.040 Positive* 10/13/98–9/19/16 0.096 ± 00.30 180
Sulfate total (mg/L) Flow (m3/s) -2.290 0.022 Negative* 10/13/98–9/19/16 15.05 ± 5.24 179
Total dissolved solids (mg/L) Flow (m3/s) 0.369 0.713 Positive 10/13/98–9/19/16 70.97 ± 24.10 174
Correlated seasonal Mann–Kendall
Streamflow (m3/s) Season (month) 0.200 0.860 Positive 1/1/98–12/31/16 38.27 ± 54.76 6940
Air temperature (°C) Season (month) 1.000 0.332 Positive 1/1/98–12/31/16 10.73 ± 9.76 6893
*Statistically significant trends at the 5% significance level.
**Statistically significant trends at the 1% significance level.
Northeastern Naturalist Vol. 26, No. 2
M.K. Shank
2019
435
distribution of Didymo throughout the watershed. Data indicate that SRP constrains
the spatial distribution, as only streams with long-term SRP median concentrations
of ≤2.7 ug/L are currently colonized by Didymo cells, which is near the threshold
suggested by Bothwell et al. (2014). Similarly, SRP concentrations appear to limit
Didymo cell density in West Branch Pine Creek and the mainstem of Pine Creek.
Tributaries and point-source discharges increase the concentration of SRP in Pine
Creek as it flows downstream, which coincides a decrease in Didymo cell density
(Fig. 1).
My observation of sites where SRP concentrations were low but Didymo was
not present suggests that other physicochemical parameters may play a role in
habitat suitability. For instance, Didymo was absent in Little Pine Creek where
mean SRP was 2.2 ± 1.7 μg/L (Fig. 1). However, this site is downstream of a riverine
impoundment with an epilimnetic release, which in combination with its large
Figure 7. Scatter plots of physicochemical variables at the sentinel monitoring location on
Pine Creek (WQN 410) that were included in trend analysis.
Northeastern Naturalist
436
M.K. Shank
2019 Vol. 26, No. 2
watershed, causes warmer summer temperatures (20.3 ± 3.1 °C) than preferred
by Didymo (Lindstrøm and Skulberg 2008, Whitton et al. 2009). Also, Little Pine
Creek has elevated sulfate concentrations (28.4 ± 13.1 mg/L), which reflect a legacy
of coal mining in the watershed. Lindstrøm and Skulberg (2008) suggested that the
sulfate concentration must be >2.5 mg/L to constitute suitable habitat for Didymo.
Additionally, there is a positive association with sulfate concentrations and Didymo
presence in Sierra Nevada streams (Rost et al. 2011). This evidence indicates that
minimum thresholds of sulfate are necessary to support Didymo stalk production;
however, no maximum preferred limit could be found in the literature. Sulfate is a
water chemistry constituent associated with abandoned mine discharge in Pennsylvania,
which also often accompanies high levels of acidity and controls solubility
of other metals (Cravotta 2008). It is possible that there is a sulfate concentration,
which, if exceeded, causes deleterious effects to Didymo. Data suggest the combination
of elevated temperature and sulfate may cause conditions in Little Pine
Creek to be unsuitable for Didymo.
Didymo mat severity in relation to physicochemical variables
Didymo mat proliferation occurred at WPIN, where long-term SRP concentrations
averaged 1.94 ± 0.95 μg/L. At this site where SRP was consistently low,
concentrations preceding sampling events did not effectively describe variation in
benthic mats. Instead, an a priori model-selection approach indicated that streamflow
flashiness and temperature in 7-d and 30-d antecedent windows, respectively,
were the best variables to predict mat severity. Notably, Didymo mats were not
present at WPIN when the 7-d Richards–Baker flashiness index values exceeded
0.25 and when 30-d mean stream temperature exceeded 15 ºC. These variables
have previously been associated with conceptual models for Didymo mat proliferation
and persistence (Cullis et al. 2012); however, determining the appropriate
time period of each variable and quantifying relationships with mat severity are
novel contributions of this research. Although previous studies have documented
associations between physicochemical variables and Didymo mat severity when
conducting instantaneous sample collections (e.g., Jackson et al. 2016), results
herein indicate the temporally variable nature of Didymo mats makes repeated
sampling efforts and/or continuous monitoring advantageous.
The Richards–Baker flashiness index outperformed other measures of flashiness
(streamflow coefficient of variation) and streamflow magnitude (i.e., number of flow
events with shear stress capable of mobilizing 32-mm diameter sediment particles)
in describing variation of Didymo mat severity. This finding suggests that variation
in streamflow with concomitant variation in depth and velocity may be more
influential in controlling benthic mats compared with high-magnitude discharge
events that result in bed mobility and occur less frequently, which corroborates
observations from streams in the Catskill Mountains of New York (Richardson et
al. 2014). Typical Didymo habitat downstream of hypolimnetic reservoir releases,
where streamflows and temperatures are stable, are often managed as sport fisheries
for salmonids. Consequently, concerns surround manipulation of dam outflows as a
Northeastern Naturalist Vol. 26, No. 2
M.K. Shank
2019
437
tool for Didymo management because they may conflict with successful salmonid
egg incubation in gravel and/or young-of-year recruitment and survival (e.g., Klauda
and Hanna 2016). However, the results herein indicate streamflow magnitude
is less important than streamflow variation in controlling benthic mats. A nuanced
approach of varying streamflow releases from dams, while staying within bounds
of acceptable salmonid habitat, may represent a potential approach for maintaining
salmonid populations while mitigating negative ecological and recreational effects
of nuisance Didymo mats. However, consideration must be given to condition of
Didymo mats and sediment content of streamflows because high streamflow events
downstream of large reservoirs often lack suspended sediments, which accelerate
degradation of stalked diatom mats (Cullis et al. 2013, Walling and Fang 2003).
My results from north-central Pennsylvania indicate that in unregulated
streams that experience seasonal flashy flows and water temperature variation,
SRP is the main factor controlling the distribution of Didymo cells. Other studies
examining similar variables in regulated streams have suggested that thresholds
supporting Didymo mats may differ between regulated and unregulated reaches.
For instance, in Rost and Fritsen’s (2014) study of 2 streams in the Sierra Nevadas,
phosphate concentrations were similar but Didymo only colonized sites
downstream of an impoundment with a hypolimnetic release. Further evidence is
present from Didymo proliferation in New York and Maryland tailwater streams,
where streamflows were regulated and temperatures rarely exceeded 16 ºC, but
median SRP varied from 4 μg/L to 10 μg/L (Klauda and Hanna 2016, Shank et al.
2016, Silldorff and Swann 2013). This finding suggests that stable discharges and
cool temperatures may allow Didymo proliferation at higher SRP concentrations.
Regulated rivers, specifically below hypolimnetic release reservoirs, represent
late-successional habitat (on the r–K continuum) that is preferred by Didymo due
to the stability in temperature and flow (Floder and Kilroy 2009, Kirkwood et al.
2009). The stasis of temperature and flow does not result in removal of Didymo
mats from the substrate, which may allow Didymo to proliferate even when SRP
concentrations are not below the threshold of 2 μg/L suggested by Bothwell et
al. (2014). Keller et al. (2017) found no association between Didymo cell presence
and water chemistry in the mid-Atlantic region; however, 4 out of 7 sites
where Didymo was present were situated downstream of hypolimnetic releases,
which, in combination with instantaneous sample collection methods, may complicate
threshold determination. Conversely, flashy flow environments result in
higher and more variable shear stress and bed mobility, which thoroughly disturb
stream habitats. These environments more frequently remove Didymo-dominated
biofilms from the substrate and simultaneously open up niches for opportunistic
algal species to colonize, thereby requiring extremely low SRP concentrations
that favor Didymo. More research is necessary to explore the potential for different
SRP thresholds in regulated versus unregulated reaches.
Pine Creek is unregulated, flashy, variable in temperature, and has quickly increasing
SRP concentrations moving downstream. As a result, late-successional
habitat is only present during natural periods of hydrologic stability and temperature
Northeastern Naturalist
438
M.K. Shank
2019 Vol. 26, No. 2
stasis. Thus, Pine Creek represents a habitat with marginal suitability for Didymo,
where mat formation is only episodic due to frequent disturbances in the form of
scouring and elevated water temperatures. Didymo mat formation in Pine Creek
is similar to Esopus Creek in New York, where stable flows and cool temperatures
were positively associated with Didymo cell density. However, the stabilizing influence
of an inter-basin aqueduct (Shandaken Portal) promotes Didymo proliferation
for a distance downstream in Esopus Creek (George and Baldigo 2015). Pine Creek,
however, is completely unregulated, and Didymo is only able to affect large reaches
when climatic conditions are favorable. For instance, in the spring of 2012, visible
Didymo mats occupied a 160-km section of the Delaware River (DRBC 2014).
Aside from occasions when all conditions remain suitable for an extended period,
we do not expect Didymo to pervade the substrate of Pine Creek, which raises
questions about the extent of impacts to the functioning of the aquatic ecosystem.
Studies have shown evidence of alterations to algal, macroinvertebrate, and fish
communities due to Didymo mat proliferation (i.e., Gillis and Lavoie 2014, James
and Chipps 2016, Kilroy et al. 2009). However, studies on Esopus Creek in New
York did not detect any severe impacts to algal, macroinvertebrate, or fish assemblages
(George and Baldigo 2015). Research should be conducted to investigate
whether infrequent, episodic Didymo mat coverage has negatively affected the
stream ecology of Pine Creek.
Changes in water chemistry
We recognize that conventional sampling protocols for microorganisms, such as
Didymo, are considered inadequate to definitively determine which taxa are truly
absent at any given point in time (Taylor and Bothwell 2014). Consequently, it is
difficult to determine if Didymo was historically absent based on previously collected
samples and/or data (Lavery et al. 2014, Spaulding and Elwell 2007). It is
perplexing, however, that when similar methods were employed to collect samples
after the first observation of Didymo in 2013 in the Pine Creek watershed, detection
of cells was easily accomplished. Data collected herein indicate range expansion in
an upstream direction on West Pine Creek during the 2014–2016 period. Similarly,
George and Baldigo (2015) found evidence of upstream range expansion in Esopus
Creek during 2009–2010. This rapid appearance and variable distribution may suggest
that Didymo populations are not in equilibrium and could undergo additional
distributional changes (Keller et al. 2017).
Based on the absence of contemporary evidence of Didymo presence in Pine
Creek despite a systematic search, the most likely explanation for Didymo colonization
is that Didymo is a native invader that may be recolonizing its former
range (Richardson et al. 2014). Regional fossilized evidence of historical Didymo
presence (i.e., Boyer 1916, 1927; Lohman 1939; Patrick and Reimer 1975) indicates
that Didymo inhabited adjacent watersheds historically, but Didymo mats
were not reported in the northeastern US prior to the early 2000s (Spaulding and
Elwell 2007). It seems plausible that changing water chemistry may have facilitated
colonization of Didymo in Pine Creek. Genetic analysis of Didymo from the
Northeastern Naturalist Vol. 26, No. 2
M.K. Shank
2019
439
mid-Atlantic indicates close relation to lineages from across the globe (Keller et al.
2017). Degradation of water quality accompanied resource extraction and industrial
activities in the watershed in the 19th and early 20th centuries. Robust datasets to
quantify this degradation do not exist from within the Pine Creek watershed, but
historic accounts describe acidic conditions from tanneries and mines and sedimentation
from widespread deforestation (Detar and Kristine 2012, PADER 1977,
Ross 1991). Widespread water quality degradation was documented throughout the
Ohio River and Lake Erie drainages in western Pennsylvania in the late 19th and
early 20th centuries (Lewis 1906, Ortmann 1909). Although somewhat qualitative,
these inventories present reliable evidence of acute levels of pollution in surface
waters sufficient to extirpate many aquatic taxa. The same historical industrial
activities and poor sanitation that caused degradation in western Pennsylvania
drainages also occurred in the Pine Creek watershed and can be assumed to have
had similar effects. Quantitative datasets from Pine Creek that span more recent
times and strengthen statistical analyses show that water chemistry parameters
with implications for Didymo habitat suitability are indeed changing. Since 1998,
sulfate concentrations declined while pH increased, which is consistent with observations
from surface waters across North America following implementation of the
Clean Air Act, indicating recovery from acidification resulting from acid deposition
(Stoddard et al. 1999). Orthophosphate shows a decreasing trend in Pine Creek,
which is consistent with broader trends in the northeastern US, as increased deposition
of N and decreased inputs of P have increased N:P ratios since 1970 (Hale et al.
2013). The continued burning of fossil fuels and modern agricultural practices are
expected to increase N concentrations, while climate-induced shifts in the timing of
snowmelt and growing season decrease P inputs to rivers, which could potentially
be responsible for further changes in water chemistry (Bothwell et al. 2014). These
converging conditions may be creating suitable Didymo habitat in streams and rivers
like Pine Creek, where it did not exist decades ago.
Current limitations and future research
The ecological paradox of Didymo occurrence—formation is restricted to nutrient
poor, often pristine settings—presents a unique paradigm for the management
of freshwater ecosystems. Moreover, the current debate surrounding its native
or non-native status further confounds our understanding of how policy makers
should respond appropriately (Elwell et al. 2014). Maryland has opted to follow
the “precautionary principle” and ban felt-sole waders and act to prevent the spread
of this microorganism (Klauda and Hanna 2016). Taylor and Bothwell (2014) appropriately
note that decontamination programs intended to prevent the spread of
microorganisms are mostly ineffective, and Vermont has repealed its ban on felt
soles (VTFWD 2016). The possible reasons for the increased nuisance behavior
of Didymo, including global climate change and anthropogenic alterations to
nutrient ratios (Bothwell et al. 2014), have not been fully supported by empirical
studies (Bergey and Spaulding 2015, Keller et al. 2017, Kunza et al. 2018). Until
this issue is resolved, the lack of clarity will make the implementation of proper
management actions problematic. Additional paleolimnological studies to place the
Northeastern Naturalist
440
M.K. Shank
2019 Vol. 26, No. 2
proliferation of Didymo into environmental context, similar to that of Lavery et al.
(2014), and regional studies to assess the distribution and association of Didymo
with physicochemical variables (e.g., Keller et al. 2017) should be encouraged to
provide clarity. Bergey and Spaulding (2015) are likely right: Didymo proliferation
and its environmental context is complicated. There may be many elements at
play, including those supported by Bothwell et al. (2014) regarding global climate
change and anthropogenic alteration of nutrients and nutrient ratios. Additional factors
that are improving water quality conditions, such as mine-drainage abatement
and enhanced industrial mitigation practices, may also affect Didymo proliferation.
Considerations must also include the possibility for human transport of this microorganism.
Future research should attempt to provide a holistic view of Didymo and
its human and environmental context so that resource managers are able to make
informed decisions.
Conclusion
This study highlights the influence of SRP on Didymo distribution. The longterm
median SRP concentration of sites that were colonized by Didymo cells was
2.7 μg/L, whereas sites in which those cells were absent had with a median SRP
concentration of 4.8 μg/L. Compared to mean values, long-term median values of
SRP may be more representative of central tendency due to the reduced effect of
high values observed during summer low flows. At WPIN, where mean SRP was
consistently less than 2μg/L, streamflow flashiness and temperature were associated with
Didymo mat severity. The examination of mat severity temporally using continuously
monitored physicochemical variables served as a novel approach that effectively
elucidated dynamics of Didymo mat proliferation in an unregulated stream.
Future research should further study the SRP thresholds that support Didymo colonization
and mat formation in unregulated streams compared to late-successional
habitat present below hypolimnetic releases, examine the ecological implications
for episodic Didymo mat coverage in unregulated streams with marginal habitat,
and investigate the historic occurrence of Didymo in the northeastern US using
paleolimnological methods.
Acknowledgments
Marina Potapova (Drexel University) performed numerous identifications of algal samples
as well as the counts and provided helpful suggestions and reviews on this manuscript.
Daniel Spooner, Kelly Maloney, Dale Honeyfield (USGS), James Shallenberger (SRBC),
and M. Potapova helped to secure funding and establish the scope of this research. Jeffrey
Zimmerman (SRBC) performed invaluable GIS work that aided analysis and clear presentation
of results. Jeffrey Butt and his colleagues at PADEP are credited with first discovery of
D. geminata in Pine Creek, which led to this research. Steven Keller (University of Vermont),
Bob Hilderbrand and Regina Trott (University of Maryland), and Jason Cessna (MDDNR)
enabled eDNA sampling. Robert Volkmar (University of Duquesne, retired) performed algal
identifications. Elizabeth Costanzo Kreger (Tioga County Conservation District) provided
historical water-quality data from Pine Creek. Dawn Hintz (SRBC) coordinated continuous
monitoring resources, and Graham Markowitz (SRBC) provided streamflow-modeling
Northeastern Naturalist Vol. 26, No. 2
M.K. Shank
2019
441
support. Katie Kline (University of Maryland Center for Environmental Science) facilitated
laboratory analysis of much of the water chemistry data included herein. Andrew Leakey,
Matthew Elsasser, Aaron Henning, Blake Maurer, Luanne Steffy, David Haklar, and John
Balay (SRBC) assisted with data collection. Pennsylvania Sea Grant and the Susquehanna
River Basin Commission provided funding for this study.
Literature Cited
Academy of Natural Sciences Pennsylvania (ANSP). 2002. Protocols for the analysis of
algal samples collected as part of the US Geological Survey National Water-Quality
Assessment Program. Report Number 02-06. The Patrick Center for Environmental
Research–Phycology Section, Philadelphia, PA. 132 pp. Available online at http://water.
usgs.gov/nawqa/protocols/algprotocol/algprotocol.pdf. Accessed 30 May 2017.
Appling, A.P., M.C. Leon, and W.H. McDowell. 2015. loadflex: Watershed flux estimates.
R package version 1.0.1. Available online at https://github.com/McDowellLab/loadflex.
Models and Tools for. Accessed 15 June 2017.
Baker, D.B., R.P. Richards, T.T. Loftus, and J.W. Kramer, 2004. A new flashiness index:
Characteristics and applications to midwestern rivers and streams. Journal of the American
Water Resources Association 40:503–522.
Barbour, M.T., J. Gerritsen, B.D. Snyder, and J.B. Stribling. 1999. Rapid bioassessment
protocols for use in streams and wadeable rivers: Periphyton, benthic macroinvertebrates,
and fish, 2nd Edition. EPA 841-B-99-002. US Environmental Protection Agency,
Office of Water, Washington, DC. 337 pp.
Bergey, E.A., and S.A. Spaulding. 2015. Didymosphenia: It’s more complicated. Bioscience
65(3):225.
Beville, S.T., G.N. Kerr, and K.F. Hughey. 2012. Valuing impacts of the invasive alga Didymosphenia
geminata on recreational angling. Ecological Economics 82:1–10.
Blanco, S., and L. Ector. 2009. Distribution, ecology, and nuisance effects of the freshwater
invasive diatom Didymosphenia geminata (Lyngbye) M. Schmidt: A literature review.
Nova Hedwigia 88:347–422.
Bothwell, M., and C. Kilroy. 2011. Phosphorus limitation of the freshwater benthic diatom
Didymosphenia geminata determined by the frequency of dividing cells. Freshwater
Biology 56:565–578.
Bothwell, M., B. Taylor, and C. Kilroy. 2014. The D. geminata story: The role of low
dissolved phosphorus in the formation of Didymosphenia geminata blooms. Diatom
Research 29(3):229–236.
Boyer, C.S. 1916. The Diatomaceae of Philadelphia and Vicinity. J.B. Lippincott Company,
Philadelphia, PA. 143 pp.
Boyer, C.S. 1927. Synopsis of North American Diatomaceae. Part II. Naviculatae, Surirellatae.
Proceedings of the Academy of Natural Sciences. Philadelphia Supplement
79:229–583.
Bray, J., J.S. Harding, C. Kilroy, P. Broady, and P. Gerbeaux. 2016. Physicochemical predictors
of the invasive diatom Didymosphenia geminata at multiple spatial scales in New
Zealand rivers. Aquatic Ecology 50(1):1–14.
Burnham, K.R., and D.R. Anderson. 2002. Model Selection and Multimodel Inference.
Springer, New York, NY. 488 pp.
Cary, S.C., K.J. Coyne, A. Rueckert, S.A. Wood, S. Kelly, C.E.C. Gemmill, C. Vieglais,
and B.J. Hicks. 2014. Development and validation of a quantitative PCR assay for the
early detection and monitoring of the invasive diatom Didymosphenia geminata. Harmful
Algae 36:63–70.
Northeastern Naturalist
442
M.K. Shank
2019 Vol. 26, No. 2
Cravotta, C.A., III. 2008. Dissolved metals and associated constituents in abandoned coalmine
discharges, Pennsylvania, USA. Part 2: Geochemical controls on constituent concentrations.
Applied Geochemistry 23:203–226.
Cullis, J., C. Gillis, M. Bothwell, C. Kilroy, A. Packman, and M. Hassan. 2012. A conceptual
model for the blooming behavior and persistence of the benthic mat-forming diatom
Didymosphenia geminata in oligotrophic streams. Journal of Geophysical Research
117(G2):G00N03.
Cullis, J.D.S., J.P. Crimaldi, and D.M. McKnight. 2013. Hydrodynamic shear removal of
the nuisance stalk-forming diatom Didymosphenia geminata. Limnology and Oceanography
Fluids and Environments 3:256–268.
Delaware River Basin Commission (DRBC). 2014. D. geminata, aka “Rock Snot”, discovered
in the non-tidal Delaware River. Available online at http://www.state.nj.us/drbc/
home/spotlight/approved/20120531_D. geminata.html. Accessed 29 March 2018.
Detar, J., and D. Kristine. 2012. Pine Creek fisheries management plan. Pennsylvania Fish
and Boat Commission, Pleasant Gap, PA. 59 pp. Available online at: http://www.fishandboat.
com/Fish/Fisheries/PineCreek/Pages/default.aspx. Accessed 20 February 2017.
Elwell, L.C., C.A. Gillis, L.A. Kunza, and M.D. Modley. 2014. Management challenges of
Didymosphenia geminata. Diatom Research 29(3):303–305.
Fitzpatrick, F.A., I.R. Waite, P.J. D’Arconte, M.R. Meador, M.A. Maupin, and M.E. Gurtz.
1998. Revised methods for characterizing stream habitat in the National Water-Quality
Assessment Program. US Geological Survey, Open–File Report 98–4052. Raleigh, NC.
77 pp.
Floder, S., and C. Kilroy. 2009. Didymosphenia geminata (Protista, Bacillariophyceae) invasion,
resistance of native periphyton communities, and implications for dispersal and
management. Biodiversity and Conservation 18:3809–3824.
George, S.D., and B.P. Baldigo. 2015. Didymosphenia geminata in the upper Esopus Creek:
Current status, variability, and controlling factors. PLoS ONE 10(7):e0130558.
Gillis, C.A., and I. Lavoie. 2014. A preliminary assessment of the effects of Didymosphenia
geminata nuisance growths on the structure and diversity of diatom assemblages of the
Restigouche River basin, Quebec, Canada. Diatom Research 29(3): 281–292.
Gillis, C.A., A.J. Dugdale, and N.E. Bergeron. 2018. Effect of discharge and habitat type on
the occurrence and severity of Didymosphenia geminata mats in the Restigouche River,
eastern Canada. Hydroecology 11(5):e1959.
Hale, R.L., J.H. Hoover, W.M. Wollheim, and C.J. Vorosmarty. 2013. History of nutrient
inputs to the northeastern United States, 1930–2000. Global Biogeochemical Cycles
27:578–591.
Hirsch, R.M. 1979. An evaluation of some record-extension techniques. Water Resources
Research 15(6):1781–1790.
Hirsch, R.M. 1982. A comparison of four streamflow record-extension techniques. Water
Resources Research 18(4):1081–1088.
Jackson, L.J., L. Corbett, and G. Scrimgeour. 2016. Environmental constraints on Didymosphenia
geminata occurrence and bloom formation in Canadian Rocky Mountain lotic
systems. Canadian Journal of Fisheries and Aquatic Sciences 73(6):964–972.
James D.A., and S.R. Chipps. 2016. Influence of Didymosphenia geminata blooms on prey
composition and associated diet and growth of Brown Trout. Transactions of the American
Fisheries Society 145:195–205.
James, D.A., M.L. Bothwell, S.R. Chipps, and J. Carreiro. 2015. Use of phosphorus to reduce
blooms of the benthic diatom Didymosphenia geminata in an oligotrophic stream.
Freshwater Science 34(4):1272–1281.
Northeastern Naturalist Vol. 26, No. 2
M.K. Shank
2019
443
Keller, S.R., R.H. Hilderbrand, M.K. Shank, and M. Potapova, 2017. Environmental DNA
genetic monitoring of the nuisance freshwater diatom Didymosphenia geminata in Eastern
North American streams. Diversity and Distributions 23(4):381–393.
Kilroy, C., and M. Bothwell. 2012. Didymosphenia geminata growth rates and bloom formation
in relation to ambient dissolved phosphorus concentration. Freshwater Biology
57:641–653.
Kilroy, C., and M. Bothwell. 2014. Attachment and short-term stalk development of
Didymosphenia geminata: Effects of light, temperature, and nutrients. Diatom Research
29(3):237–248.
Kilroy, C. and P. Novis. 2018. Is Didymosphenia geminata an introduced species in New
Zealand? Evidence from trends in water chemistry, and chloroplast DNA. Ecology and
Evolution 8(2):904–919.
Kilroy, C., and M. Unwin. 2011. The arrival and spread of the bloom-forming, freshwater
diatom, Didymosphenia geminata, in New Zealand. Aquatic Invasions 6(3):249–262.
Kilroy C., S.T. Larned, and B.J.F. Biggs. 2009. The non–indigenous diatom Didymosphenia
geminata alters benthic communities in New Zealand rivers. Freshwater Biology
54(9):1990–2002.
Kirkwood, A., L. Jackson, and E. McCauley. 2009. Are dams hotspots for Didymosphenia
geminata blooms? Freshwater Biology 54:1856–1863.
Klauda, R.J., and K.V. Hanna. 2016. Didymosphenia geminata infestation in Maryland:
Reactions and responses by the Maryland Department of Natural Resources 2008–2014.
Maryland Department of Natural Resources, Annapolis, MD. 36 pp.
Kunza, L.A. C.A. Gillis, J.Z. Haueter, J.N. Murdock, and J.M. O’Brien. 2018. Declining
phosphorus as a potential driver for the onset of Didymosphenia geminata mats in
North American rivers. River Research and Applications 34:1105–1110. DOI:10.1002/
rra.3332.
Lavery, J., J. Kurek, K. Ruhland, C. Gillis, M. Pisaric, and J. Smol. 2014. Exploring the
environmental context of recent Didymosphenia geminata proliferation in Gaspesie,
Quebec, using paleolimnology. Canadian Journal of Fisheries and Aquatic Sciences
71(4):616–626.
Letham, B., A. Martindale, R. Macdonald, E. Guiry, J. Jones, and K.M. Ames. 2016. Postglacial
relative sea-level history of the Prince Rupert area, British Columbia, Canada.
Quaternary Science Reviews 153:156–191.
Lewis, S.J. 1906. Quality of water in the upper Ohio River Basin and at Erie, PA. US Geological
Survey Water-Supply and Irrigation Paper 161. US Government Printing Office,
Washington, DC. 114 pp. Available online at https://pubs.usgs.gov/wsp/0161/report.pdf.
Lindstrøm, E.A., and O. Skulberg. 2008. Didymosphenia geminata: A native diatom species
of Norwegian rivers coexisting with the Atlantic salmon. Pp. 35–40, In M.L. Bothwell
and S.A. Spaulding (Eds.). Proceedings of the 2007 International Workshop on Didymosphenia
geminata. Canadian Technical Report on Fisheries and Aquatic Sciences
Fisheries and Oceans Canada, Nanimo, BC, Canada. 96 pp.
Lohman, K.E. 1939. Pleistocene diatoms from Long Island, New York. US Geological Survey
Professional Paper 189:229–235.
Lorenz, D., R. Runkel, and L. De Cicco. 2015. rloadest: River Load Estimation. R package
version 0.4.3.
Ortmann, A.E. 1909. The destruction of fresh-water fauna in Western Pennsylvania. Proceedings
of the American Philosophical Society 48(191):90–110.
Northeastern Naturalist
444
M.K. Shank
2019 Vol. 26, No. 2
Patrick, R., and C.W. Reimer. 1975. Diatoms of the United States, Volume 1. Academy of
Natural Sciences of Philadelphia. Monograph Number 13, Philadelphia, PA. 688 pp.
Pennsylvania Department of Environmental Resources (PADER). 1977. Pine Creek watershed
report on mine-drainage pollution. Bureau of Water Quality Management, Harrisburg,
PA. 25 pp.
Pennsylvania State Climatologist. 2017. Daily weather data for Williamsport, PA. Available
online at http://climate.psu.edu/data/ida/index.php?t=3andx=faa_dailyandid=KIPT. Accessed
25 February 2017.
Pite, D.P, K.A. Lane, A.K. Hermann, S.A. Spaulding, and B.P. Finney. 2009. Historical
abundance and morphology of Didymosphenia species in Naknek Lake, Alaska. Acta
Botanica Croatica 68(2):183–197.
Pohlert, T. 2016. trend: Non-parametric trend tests and change-point detection. R package
version 0.2.0. Available online at https://CRAN.R–project.org/package=trend. Accessed
17 February 2017.
Potapova, M. 2010. Diatoms of north-central Pennsylvania. Final Report for Grant Agreement
WRCP–08286. Academy of Natural Sciences, Philadelphia, PA. 40 pp.
R Core Team, 2016. R: A language and environment for statistical computing. R Foundation
for Statistical Computing, Vienna, Austria. Available at https://www.R–project.org/.
Accessed 1 February 2017.
Richardson, D.C., I.A. Oleksy, T.J. Hoellein, D.B. Arscott, C.A. Gibson, and S.M. Root.
2014. Habitat characteristics, temporal variability, and macroinvertebrate communities
associated with a mat-forming nuisance diatom (Didymosphenia geminata) in Catskill
mountain streams, New York. Aquatic Sciences 76:553–564.
Ross, R.M. 1991. The effect of acid mine-drainage and related sources of pollution on
aquatic macroinvertebrate life in Pine Creek. Pine Creek Headwaters Protection Group,
Wellsboro, PA. 14 pp.
Rost, A.L., and C.H. Fritsen. 2014. Influence of a tributary stream on benthic communities
in a Didymosphenia geminata-impacted stream in the Sierra Nevada, USA. Diatom
Research 29(3):249–257.
Rost, A.L., C.H. Fritsen, and C.J. Davis. 2011. Distribution of freshwater diatom Didymosphenia
geminata in streams in the Sierra Nevada, USA, in relation to water chemistry
and bedrock geology. Hydrobiologia 665:157–167.
Ruzycki, E.M., R.P. Axler, G.E. Host, J.R. Henneck, and N.R. Will. 2014. Estimating sediment
and nutrient loads in four western Lake Superior Streams. Journal of the American
Water Resources Association 50(5):1138–1154.
Shank, M.K., M. Potapova, K. Maloney, D. Honeyfield, and D.E. Spooner. 2016. Didymosphenia
geminata in Pennsylvania: An investigation of current and historic distribution,
habitat suitability, and nutrient content. Susquehanna River Basin Commission, Harrisburg,
PA. 58 pp. Available online at http://seagrant.psu.edu/topics/invasive-species/
research/didymosphenia-geminata-pennsylvania-investigation-current-and. Accessed 1
February 2017.
Silldorff, E.L., and M.M. Swann. 2013. Observational and experimental work with Didymosphenia
geminata in the lower Delaware River: A report to the Pennsylvania SeaGrant.
Delaware River Basin Commission, West Trenton, NJ. 42 pp. Available online at http://
seagrant.psu.edu/topics/invasive–species/research/nutrient–thresholds–didymosphenia–
geminata–blooms–and–stalk. Accessed 22 January 2017.
Spaulding, S., and L. Elwell. 2007. Increase in nuisance blooms and geographic expansion
of the freshwater diatom Didymosphenia geminata. Open-file Report 2007-1425. US
Geological Survey, Reston, VA. 42 pp.
Northeastern Naturalist Vol. 26, No. 2
M.K. Shank
2019
445
Stets, E.G., V.J. Kelly, W. Broussard, T.E. Smith, and C.G. Crawford. 2012. Century-scale
perspective on water quality in selected river basins of the conterminous United States.
Scientific Investigations Report 2012–5225. US Geological Survey, Reston, VA. 120 pp.
Stoddard, J.L., D.S Jeffries, A. Lukewille, T.A. Clair, P.J. Dillon, C.T. Driscoll, M. Forsius,
M. Johannessen, J.S. Kahl, J.H. Kellogg, A. Kemp, J. Mannio, D.T. Monteith, P.S. Murdoch,
S. Patrick, A. Rebsdorf, B.L. Skjelkvale, M.P. Stainton, T. Traaen, H. van Dam,
K.E. Webster, J. Wieting, and A. Wilander. 1999. Regional trends in aquatic recovery
from acidification in North America and Europe. Nature 401:575–578.
Susquehanna River Basin Commission (SRBC). 2017. Standard operating procedure: Remote
water quality monitoring network. Susquehanna River Basin Commission, Harrisburg,
PA. 61 pp. Available online at http://mdw.srbc.net/remotewaterquality/methods.
htm. Accessed4 August 2017.
Taylor, B.W., and M. L. Bothwell. 2014. The origin of invasive microorganisms matters
for science, policy, and management: The case of Didymosphenia geminata. BioScience
64(6):531–538.
US Environmental Protection Agency (USEPA). 2017. Storage and retrieval and waterquality
exchange (STORET). Available online at http://www.epa.gov/storet/. Accessed
25 February 2017.
US Geological Survey (USGS). 2016. The StreamStats program for Pennsylvania. Available
online at https://water.usgs.gov/osw/streamstats/pennsylvania.html. Accessed 14
December 2016.
Vermont Fish and Wildlife Department (VTFWD). 2016. Felt-soled wader ban is repealed.
Available online at http://vtfishandwildlife.hosted.civiclive.com/cms/One.aspx?portalId
=73163&pageId=4278738. Accessed 29 March 2018.
Walling, D.E., and D. Fang. 2003. Recent trends in the suspended sediment loads of the
world’s rivers. Global and Planetary Change 39:111–126.
Whitton, B., N. Ellwood, and B. Kawecka. 2009. Biology of the freshwater diatom Didymosphenia:
A review. Hydrobiologia 630:1–37.